Detectability of Surface Biosignatures for Directly-Imaged Rocky Exoplanets
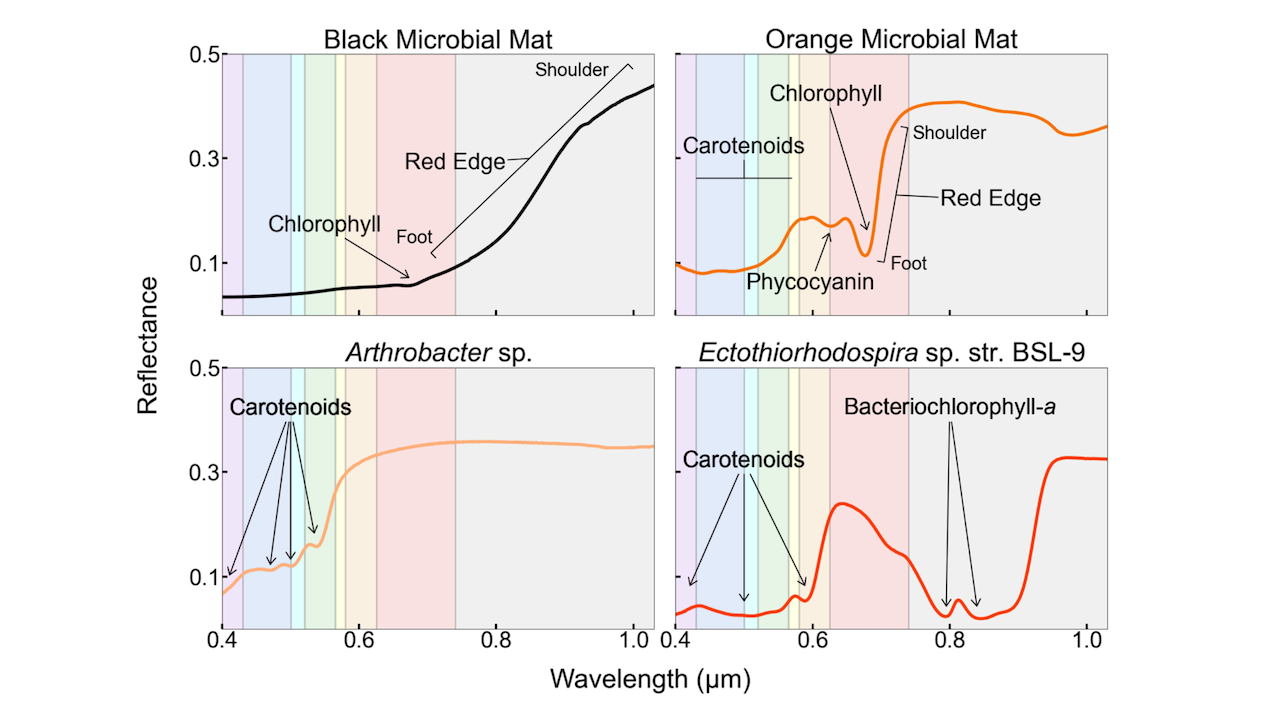
Modeling the detection of life has never been more opportune. With next generation space telescopes, like the currently developing Habitable Worlds Observatory (HWO) concept, we will begin to characterize rocky exoplanets potentially similar to Earth.
However, currently, few realistic planetary spectra containing surface biosignatures have been paired with direct imaging telescope instrument models. Therefore, we use a HWO instrument noise model to assess the detection of surface biosignatures affiliated with oxygenic, anoxygenic, and nonphotosynthetic extremophiles.
We pair the HWO telescope model to a 1-D radiative transfer model to estimate the required exposure times necessary for detecting each biosignature on planets with global microbial coverage and varying atmospheric water vapor concentrations. For modeled planets with 0% – 50% cloud coverage, we determine pigments and the red edge could be detected within 1,000 hours (100 hours) at distances within 15 pc (11 pc). However, tighter telescope inner working angles (2.5 lambda/D) would allow surface biosignature detection at further distances.
Anoxygenic photosynthetic biosignatures could also be more easily detectable than nonphotosynthetic pigments and the photosynthetic red edge when compared against a false positive iron oxide slope. Future life detection missions should evaluate the influence of false positives on the detection of multiple surface biosignatures.
Images of the four microbes used in this study. Black and orange microbial mats (top left and right, respectively) are oxygenic photosynthetic microbial communities from the Fryxell Basin of Taylor Valley, Antarctica. Scale bars in both images are 10 cm. Both spectra and images were collected in the field as part of the database from Borges et al. (2023). Arthrobacter sp. and 42 Ectothiorhodospira sp. str. BSL-9 (bottom left and right, respectively) are nonphotosynthetic and anoxygenic photosynthetic microbes grown on 2.5 cm diameter filters in the lab as part of work conducted in Hegde et al. (2015). Images and spectra are from Hegde et al. (2015). — astro-ph.EP
Schuyler R. Borges, Gabrielle G. Jones, Tyler D. Robinson
Comments: in press; as-accepted version, prior to journal editing
Subjects: Earth and Planetary Astrophysics (astro-ph.EP)
Cite as: arXiv:2404.16126 [astro-ph.EP] (or arXiv:2404.16126v1 [astro-ph.EP] for this version)
Related DOI:
https://doi.org/10.1089/ast.2023.0099
Focus to learn more
Submission history
From: Tyler Robinson
[v1] Wed, 24 Apr 2024 18:31:17 UTC (2,705 KB)
https://arxiv.org/abs/2404.16126
Astrobiology