Nanosized Blocks Spontaneously Assemble In Water To Create Tiny Floating Checkerboards
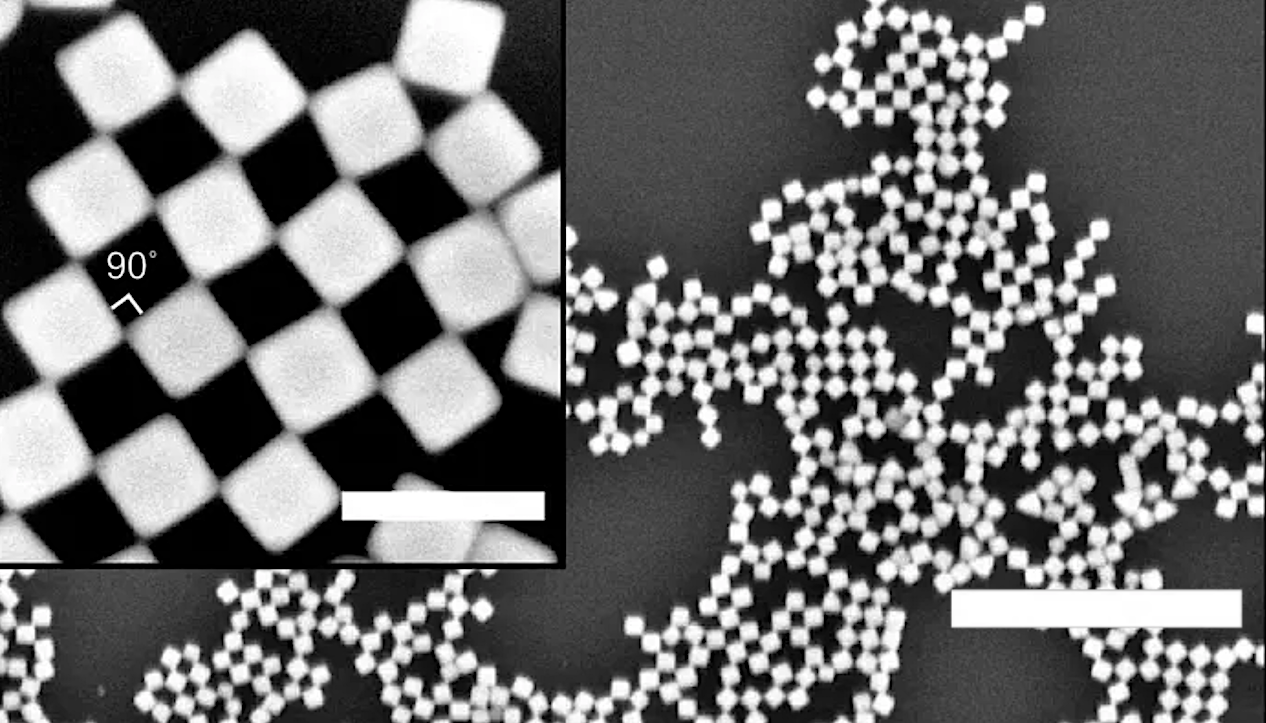
Researchers have engineered nanosized cubes that spontaneously form a two-dimensional checkerboard pattern when dropped on the surface of water. The work, published in Nature Communications, presents a simple approach to create complex nanostructures through a technique called self-assembly.
“It’s a cool way to get materials to build themselves,” said study co-senior author Andrea Tao, a professor in the Aiiso Yufeng Li Family Department of Chemical and Nano Engineering at the University of California San Diego. “You don’t have to go into a nanofabrication lab and do all these complex and precise manipulations.”
Each nanocube is composed of a silver crystal with a mixture of hydrophobic (oily) and hydrophilic (water-loving) molecules attached to the surface. When a suspension of these nanocubes is introduced to a water surface, they arrange themselves such that they touch at their corner edges. This arrangement creates an alternating pattern of solid cubes and empty spaces, resulting in a checkerboard pattern.
The self-assembly process is driven by the surface chemistry of the nanocubes. A high density of hydrophobic molecules on the surface brings the cubes together to minimize their interaction with water. Meanwhile, the long chains of hydrophilic molecules cause enough repulsion to create voids between the cubes, creating the checkerboard pattern.
To fabricate the structure, researchers applied drops of the nanocube suspension onto a petri dish containing water. The resulting checkerboard can be easily transferred to a substrate by dipping the substrate into the water and slowly withdrawing it, allowing the nanostructure to coat it.
This study stems from a collaborative effort between multiple research groups that are part of the UC San Diego Materials Research Science and Engineering Center (MRSEC). The work featured a synergistic combination of computational and experimental techniques. “We’ve built a continuous feedback loop between our computations and experiments,” said Tao. “We used computer simulations to help us design the materials at the nanoscale and predict how they will behave. We also used our experimental results in the lab to validate the simulations, fine tune them and build a better model.”
In designing the material, researchers chose silver crystal nanocubes due to the Tao lab’s expertise in their synthesis. Determining the optimal surface chemistry required extensive computational experimentation, which was led by Gaurav Arya, a professor in the Department of Mechanical Engineering and Materials Science at Duke University and co-senior author of the study.
The simulations identified the best molecules to attach to the nanocubes and predicted how the cubes would interact and assemble on the water surface. The simulations were iteratively refined using experimental data obtained by Tao’s lab. Electron microscopy performed by the lab of study co-author Alex Frañó, a professor in the Department of Physics at UC San Diego, confirmed the formation of the desired checkerboard structures.
Tao envisions applications for the nanocube checkerboard in optical sensing. “Such a nanostructure can manipulate light in interesting ways,” she explained. “The spaces between the cubes, particularly near the corner edges where the cubes connect, can act as tiny hotspots that focus or trap light. That could be useful for making new types of optical elements like nanoscale filters or waveguides.”
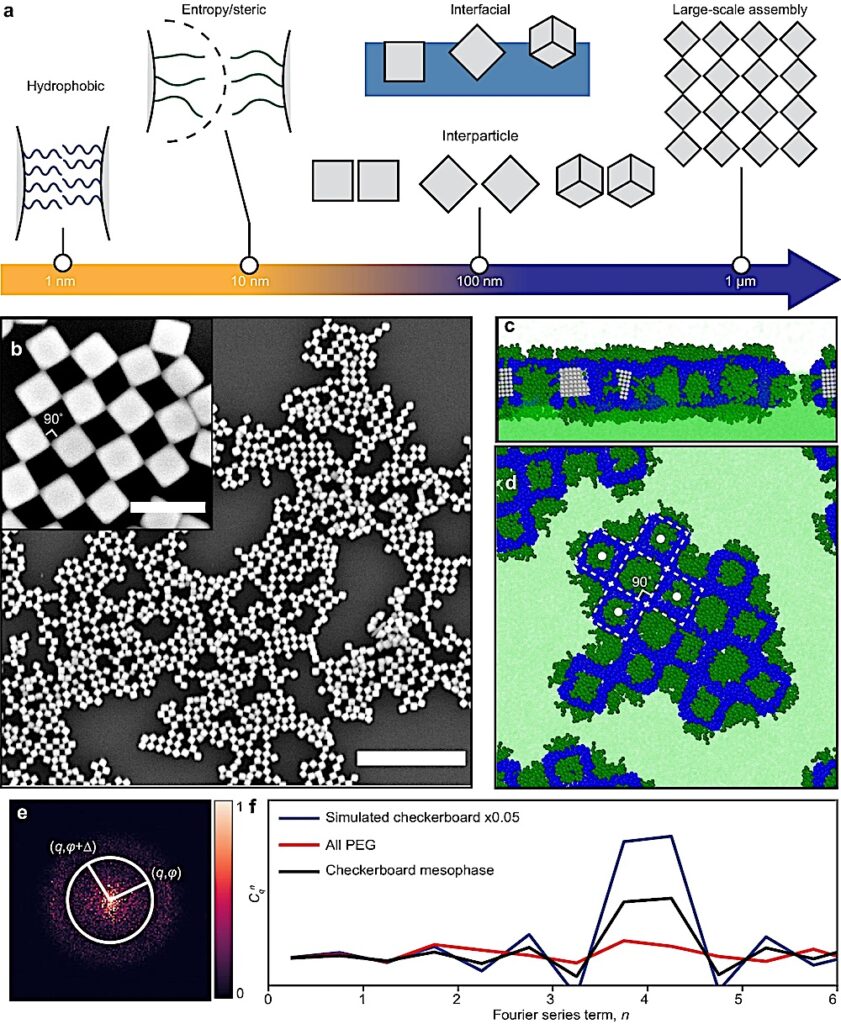
a Design strategies operating at different scales were harnessed together to achieve the assembly. b SEM image of the mesophase constructed with edge–edge connected checkerboard lattice obtained with Ag NCs post-synthetically modified with a feedstock mixture of 50 µM PEG20k-SH and 6 µM C16-SH. Scale bar = 500 nm, inset = 100 nm. c, d Simulation snapshots of the assembled NC checkerboard lattice at an interface with side (c) and top (d) views. The PEG20k-SH, C16-SH, and water are represented by dark green, blue, and light green, respectively. e A 2D Fourier transform magnitude of an SEM image over a larger field of view (4.5 µm x 4.5 µm) was used to quantify the extent of local order by evaluating the angular cross-correlation (ACC) function Cq(Δ) between the intensity at two points separated by an angular separation, Δ, at a given q value inversely proportional to the spatial length scale of the order being probed. The details of this analysis are provided in Supplementary Figs. 1 and 2. f A second Fourier transform is taken on the ACC function Cq(Δ) to extract the symmetry order n of the function with respect to Δ, Cnq (Supplementary Note 3). An increase in the four-fold symmetry term n = 4 in samples with PEG:C16 = 50 μM:6 µM ligand feedstock (green) indicates the presence of checkerboard order compared to the all-PEG sample (red). For reference, the blue line shows Cnq evaluated for a simulated perfect checkerboard pattern, scaled down by 0.05 for easy comparison. Source data are provided as a Source Data file. — Nature Communications
The researchers plan to explore the optical properties of the checkerboard in future studies.
Paper: “Self-assembly of nanocrystal checkerboard patterns via non-specific interactions.” Co-authors include Yufei Wang, Rourav Basak, Yu Xie, Dong Le, Alexander D. Fuqua, Wade Shipley and Zachary Yam, UC San Diego; and Yilong Zhou and Quanpeng Yang, Duke University.
*These authors contributed equally.
This work was supported by the National Science Foundation, UC San Diego Materials Research Science and Engineering Center (DMR-2011924). The work was performed in part at the San Diego Nanotechnology Infrastructure (SDNI) at UC San Diego, a member of the National Nanotechnology Coordinated Infrastructure, which is supported by the National Science Foundation (grant ECCS-2025752).
Self-assembly of nanocrystal checkerboard patterns via non-specific interactions, Nature Communications (open access)
Astrobiology, SynBio, Nanotechnology,