The Light Of TRAPPIST-1 b Reveals New Insights On The Planet’s Nature
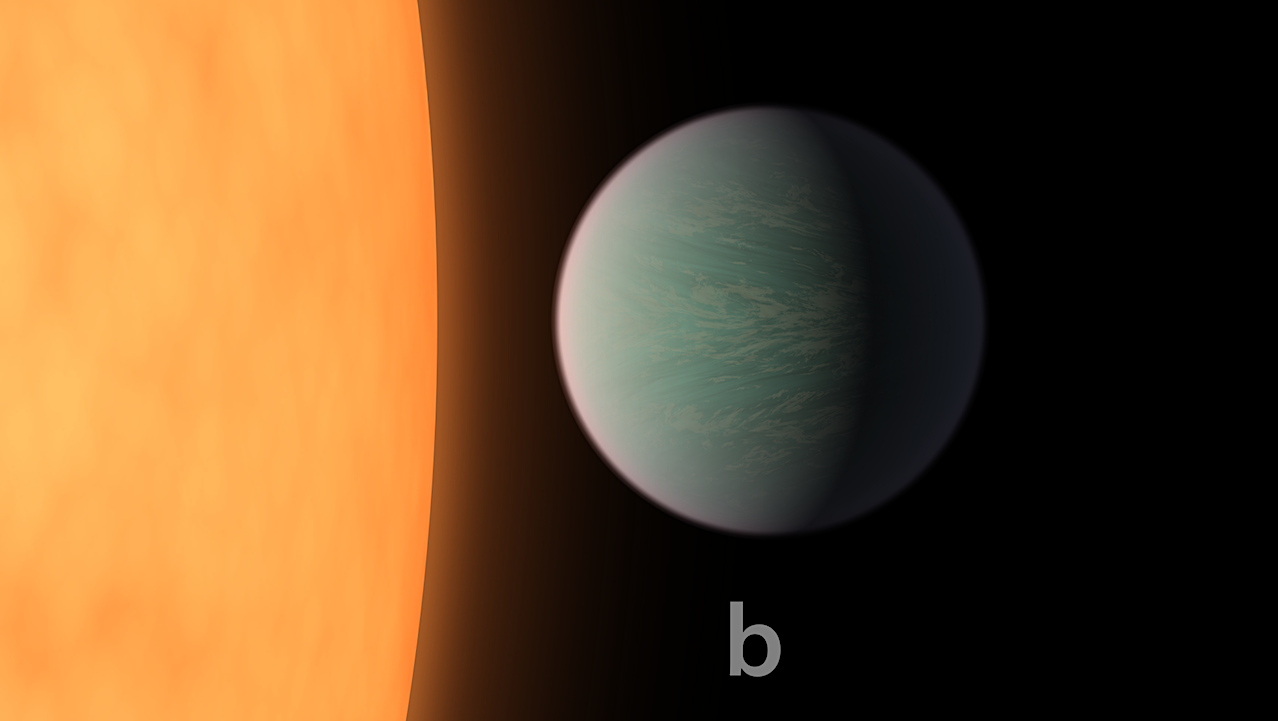
New TRAPPIST-1 observations with JWST underscore the complexities of confirming a planet’s atmosphere using only broadband thermal emission data. This insight takes on added significance with the newly approved “Rocky Worlds” observation program by Space Telescope Science Institute (STScI) which plans to apply this very method to study numerous rocky exoplanets orbiting cool stars.
The James Webb Space Telescope (JWST) is revolutionizing the study of exoplanets (planets orbiting stars other than the Sun), notably by enabling detailed spectroscopic studies of small rocky planets, but only if they orbit nearby ‘red dwarfs’, the smallest, least massive and coldest stars. At the top of its list of targets is the very low-mass red dwarf TRAPPIST-1, whose astonishing system of seven rocky planets the size of Earth, including three located in the star’s habitable zone, was discovered in 2017 by an international team led by ULiège astronomer Michaël Gillon.
The innermost planet, TRAPPIST-1 b, was recently observed in depth by JWST in the mid-infrared, a type of light to which our eyes are not sensitive. An international team of researchers has just published in Nature Astronomy a complete analysis of all the mid-infrared data collected on TRAPPIST-1 b, with the aim of determining whether this planet has an atmosphere.
‘Planets orbiting red dwarfs are our best chance of studying for the first time the atmospheres of temperate rocky planets, those that receive stellar fluxes between those of Mercury and Mars’, explains Elsa Ducrot, co-lead author of the study and assistant astronomer at the Commissariat aux Énergies Atomiques (CEA) in Paris, France. ‘The TRAPPIST-1 planets provide an ideal laboratory for this ground-breaking research.
A previous observation with JWST measured TRAPPIST-1 b’s infrared emission at 15 microns and suggested that a thick, CO2-rich atmosphere was unlikely (Greene et al., 2023). This conclusion was based on the fact that CO2 strongly absorbs radiation at this wavelength, which would have significantly reduced the observed flux if such an atmosphere were present.
The study proposed that the measurement was most consistent with a “dark bare rock” scenario— a planet without an atmosphere and a dark surface that absorbs nearly all incoming starlight. However, a single measurement at one wavelength was insufficient to rule out all potential atmospheric scenarios
In this new study, the authors expanded on this work by measuring the planet’s flux at another wavelength, 12.8 microns. They conducted a global analysis of all available JWST data and compared these observations with surface and atmospheric models to identify the scenario that best matches the data.
Emission to the rescue
The method most used to determine whether an exoplanet has an atmosphere – transit transmission spectroscopy – involves observing its ‘transits’, i.e. when it passes in front of its host star at different wavelengths and detecting and measuring the tiny fraction of the light emitted by the star in our direction that is absorbed by its atmosphere, which is an indicator of its chemical composition.
‘However, very low-mass red dwarfs pose a problem in this respect,’ explains Professor Michaël Gillon (ULiège), author of the study. ‘Their surface is not homogeneous, and this inhomogeneity can pollute the transmission spectrum of transiting planets and mimic atmospheric characteristics.’ Such a phenomenon has been observed on several occasions with the JWST during the observation of transits of planets around red dwarfs.
One solution to overcome this stellar contamination and still get information about the presence (or absence) of an atmosphere is to directly measure the planet’s heat by observing a drop in flux as the planet passes behind the star (an event called occultation). By observing the star just before and during the occultation, we can deduce the amount of infrared light coming from the planet.
‘Emission quickly became the preferred method for studying rocky exoplanets around red dwarfs during the first two years of JWST,’ explains Pierre Lagage, co-lead author of the study and head of the astrophysics department at the Commissariat aux Énergies Atomiques (CEA) in Paris, France. ‘For the TRAPPIST-1 planets, the first information comes from emission measurements, because it is still difficult to disentangle the atmospheric and stellar signals in the transit.
Reflecting this growing interest, the Space Telescope Science Institute (STScI), that manages JWST operations, recently approved a 500 hours Director Discretionary Time (DDT) program called ‘Rocky Worlds‘ to investigate the atmospheres of terrestrial exoplanets around nearby M-dwarf stars using exactly the same approach as the authors, via occultation observations, but at 15 microns only.
The results of the study are not very consistent with the ‘dark, bare surface’ scenario suggested by Greene et al. 2023. The authors found that a not-so-grey bare surface composed of ultramafic rocks (volcanic rocks enriched in minerals) better explained the data.
Alternatively, they were able to show that an atmosphere with a large amount of CO2 and haze could also explain the observations. This was a surprising result, since a CO2-rich atmosphere seemed incompatible with the strong emission at 15 microns. However, haze can radically change the situation: it can effectively absorb starlight and make the upper atmosphere warmer than the lower layers, creating what is known as a ‘thermal inversion’, like the Earth’s stratosphere. This inversion causes the CO2 to emit light rather than absorb it, resulting in a higher flux at 15 microns than at 12.8 microns.
“These thermal inversions are quite common in the atmospheres of Solar system bodies, perhaps the most similar example being the hazy atmosphere of Saturn’s moon Titan. Yet, the chemistry in the atmosphere of TRAPPIST-1b is expected to be very different from Titan or any of the Solar system’s rocky bodies and it is fascinating to think we might be looking at a type of atmosphere we have never seen before” explains Dr. Michiel Min from SRON Netherlands Institute for Space Research.
The authors note, however, that this atmospheric model, while consistent with the data, remains less likely than the bare rock scenario. Its complexity and the questions relating to haze formation and long-term climate stability on TRAPPIST-1 b make it a difficult model to implement. Future research, including advanced 3D modelling, will be needed to explore these issues. More generally, the team stresses the difficulty of determining with certainty a planet’s surface or atmospheric composition using only emission measurements in a few wavelengths, while highlighting two convincing scenarios that will be explored in greater detail with the next observations of TRAPPIST-1 b.
What’s next?
‘Although both scenarios remain viable, our recent observations of TRAPPIST-1 b’s phase curve – which tracks the flow of the planet throughout its orbit – will help to solve the mystery’, says Professor Michaël Gillon, who co-directs the new JWST program with Dr Elsa Ducrot. She adds: ‘By analyzing the efficiency with which heat is redistributed on the planet, astronomers can deduce the presence of an atmosphere. If an atmosphere exists, the heat should be distributed from the day side of the planet to its night side; without an atmosphere, the redistribution of heat would be minimal.”
So we should soon know more about the presence or absence of an atmosphere around TRAPPIST-1’s inner planet.
Combined analysis of the 12.8 and 15 μm JWST/MIRI eclipse observations of TRAPPIST-1 b, Nature
Astrobiology