Congressional Testimony By Natalie Batalha: Early Science Results for Transiting Exoplanets Observed by JWST
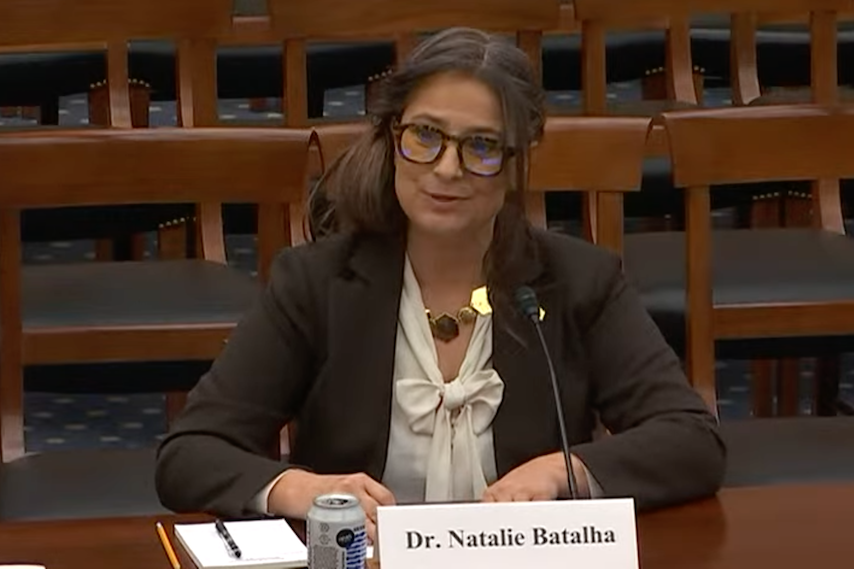
JWST is ushering in a new epoch of exoplanet science that will rewrite textbooks. Progress will stem largely from the characterization of exoplanet atmospheres enabled by JWST technology. Herein, I will focus on the method of transmission spectroscopy. I note that coronagraphic observations from JWST will also provide significant contributions to our understanding of planet formation and evolution.
Background
To date, thousands of planets orbiting other stars in the Galaxy have been identified. Most were discovered using the Transit Method which involves precise monitoring of the brightness of stars to search for the dimming of light that occurs when a planet eclipses (or “transits” in front of) its star, blotting out a fraction of the light as seen from the telescope’s vantage point (Figure 1). The dimming repeats like clockwork, once every orbit. The amount of dimming tells us the radius of the star and the time between dimming events tells us the orbital period. Johannes Kepler taught us in the 1600’s that the orbital period is related to the distance between the star and the planet which is how we know if a planet is orbiting in the star’s Habitable Zone.

Figure 1. The Transit Method for detecting exoplanets is based on precise measurements of stellar brightness. Planets with edge- on orbits relative to our line of sight eclipse their star producing a dimming of the starlight that repeats once each orbit. During the planet’s transit across the face of the star, some light will pass through the planet’s atmosphere (here exaggerated by the gray annulus). Atoms and molecules in the atmosphere will imprint a chemical fingerprint on the light. (Credit: L. Hustak, STScI)
This is the method employed by NASA space missions like Kepler (2009 – 2017) and TESS (2018 – present). Kepler taught us that planets are common in the Galaxy. On average, every sun-like star has at least one planet and more than 10 billion planets are terrestrial-sized and orbit in the Habitable Zone where liquid water could pool on the surface under the right conditions.
Kepler also taught us that the diversity of planets in the Galaxy far exceeds the diversity of planets in our Solar System. We know of worlds with lava oceans, two rising suns, lock-step orbits, and even comet-like tails. We also know of planets intermediate in size to the terrestrials and gas giants in our own Solar System. Dubbed “super-earths” or “mini-neptunes” (occasionally even “water worlds”), these are the most common types of planets known to us and yet we have no equal in our solar system. It behooves us to understand the physical processes that lead to such diversity in the Galaxy and how those processes impact planetary habitability.
The study of demographics has been limited to the examination of bulk planet properties – properties like the total mass and radius gleaned from detection surveys. Applying the method of “transmission spectroscopy” to hundreds of exoplanets will yield a new lens on planetary diversity. JWST is uniquely suited to this task for nearby exoplanets (like those identified by NASA’s TESS Mission). From JWST data collected over the next decade, we will gain a much deeper understanding of how planets form and evolve, and we will be better equipped to identify the most likely abodes of life with future missions.
Transmission Spectroscopy
Transiting planets are especially valuable for scientific studies. Kepler and TESS observe transits in, effectively, “white light” whereby many colors are combined together. JWST is observing exoplanet transits too. But thanks to ultra-stable spectrometers, JWST observes transits in hundreds of infrared colors simultaneously (Figure 2).

Figure 2. JWST observes planetary transits in hundreds of infrared colors (wavelengths) simultaneously. Shown here are 25 transits, stacked vertically, each representing a different wavelength. The Carbon Dioxide (CO2) molecule, for example, absorbs light preferentially at 4.3 ÎĽm (second transit from the top). A transiting planet with CO2 molecules in its atmosphere will block out more starlight at 4.3 ÎĽm than at other colors. (Image Credit: Zafar Rustamkulov, JHU)
When planets eclipse their host star, some of the starlight passes through the planet’s thin atmosphere on its way to Earth’s telescopes. The atmosphere imprints a chemical fingerprint on the light since atoms and molecules absorb starlight at distinct colors. In effect, the planet blocks a slightly different fraction of light depending on the color and depending on which molecules are present in the atmosphere. The spectrometers aboard JWST allow us to measure the amount of light blocked at each color thereby building up an atmospheric “spectrum.”
This method of studying planetary atmospheres is called “transmission spectroscopy,” and it has been employed by the Hubble Space Telescope and large ground-based telescopes on a small number of (mostly giant) planets. JWST is extending the capability and increasing sensitivity by collecting more photons in a stable environment across a broader range of infrared wavelengths where a variety of molecules generate strong absorption features.
Pre-launch simulations predicted that JWST data will enable atomic and molecular abundance measurements, atmospheric temperature and pressure determinations, an understanding of heavy element enrichment in planetary systems, investigation of cloud dynamics and haze production, and studies of photoevaporation and photochemistry for hundreds of planets down to super-earth and sub-neptune sizes. Pre-launch simulations also predicted that JWST will be able to detect the presence of atmospheres on terrestrial-size planets orbiting M-type stars, including a number of planets that orbit in the Habitable Zone (e.g. those orbiting TRAPPIST-1).
Early Science with JWST
In 2016, the exoplanet science community started an open-science initiative to discuss strategies for the first exoplanet observations with JWST. This effort resulted in Early Release Science Program ERS-1366. It has grown to include over 300 scientists working collaboratively. The program aims to test all of the JWST observing modes capable of performing transmission spectroscopy by observing three giant planets in short-period orbits (i.e. “hot jupiters”): WASP- 39b, WASP-18b, and WASP-43b. Each planet had previously been observed by the Hubble Space Telescope, and each showed some evidence of atmospheric absorption. The goal of the program is to provide representative data to the community together with analysis tools and best practices.
Herein, I report on early science results for WASP-39b. WASP-39 is a sun-like star approximately 700 light-years away toward the constellation Virgo. The transiting exoplanet is 30% larger than Jupiter with a mass comparable to that of Saturn. It orbits the star once every four days at a distance that’s 8 times closer than Mercury is to our Sun. Four transits of the exoplanet have been observed, each with a different instrument mode.
A transmission spectrum is constructed by measuring the amount of light blocked during eclipse at each infrared color. Figure 3 shows the spectrum from NIRSpec PRISM. The most prominent feature near 4.3 microns is due to the absorption of starlight by Carbon Dioxide (CO2) molecules – the first robust detection of CO2 in an exoplanet atmosphere [1]. CO2 is present in all solar system planets that have atmospheres. It’s also the primary background gas that we expect to detect in terrestrial-size exoplanets. Moreover, it’s a very sensitive tracer of the overall heavy element enrichment in a planetary atmosphere.
Other science discoveries gleaned from the transmission spectra of WASP-39 b include:
- The identification of multiple chemical species in the atmosphere, including sodium (Na), potassium (K), four independent water (H2O) features, carbon dioxide (CO2), and carbon monoxide (CO).
- Evidence of clouds. The data suggest that the cloud deck is not one uniform blanket. To fit the spectral features, higher complexity in the cloud deck is required as seen on solar system giant planets.
- The first detection of sulfur dioxide (SO2), a molecule produced from chemical reactions triggered by high-energy light from the planet’s parent star. On Earth, the protective ozone layer in the upper atmosphere is created in a similar way (Figure 4).
More than a dozen scientific studies using these data are in progress. We will be able to quantify chemical abundances, study disequilibrium processes, vertical mixing and photochemistry, search for additional molecules, and constrain the temperature and pressure structure of the atmosphere.
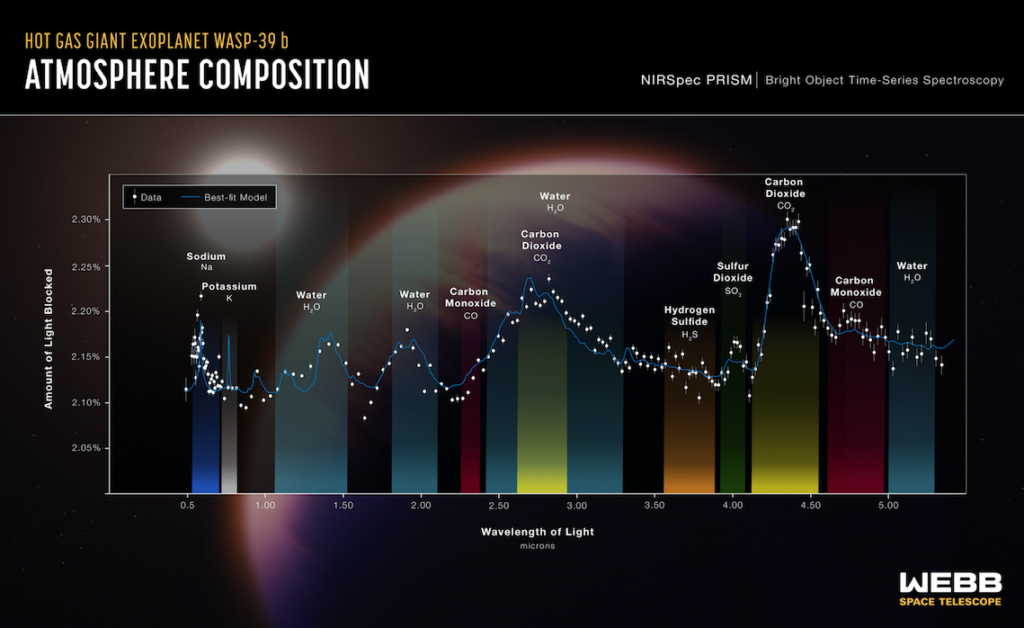
Figure 3. The atmospheric spectrum of the exoplanet WASP-39b from JWST’s NIRSpec PRISM instrument is generated by measuring the amount of starlight blocked by the planet (y-axis) at each wavelength (x-axis). The measurements are shown here as white circles. The shaded regions in the background mark wavelengths impacted by a variety of atoms and molecules. The rise and fall of the data points align with these regions. JWST data reveal the presence of Sodium, Potassium, Water, Carbon Dioxide, Sulfur Dioxide, and Carbon Monoxide in the atmosphere of WASP-39b. (Image Credit: STScI)
Instrument Performance:
The WASP-39 b observations allow us to independently investigate the performance of the JWST instruments. The open-science nature of the Transiting Exoplanet Early Release Science Team allows us to apply independent analysis pipelines to the data for robust intercomparison of performance benchmarks. Observing the same exoplanet with multiple instruments at overlapping wavelengths allows us to perform consistency checks. We find:
- The telescope and its instruments are performing at or better than pre-launch expectations. This finding is in agreement with the commissioning test on the exoplanet HAT-P-14 b. [2]
- JWST yields unprecedented precision and stability in spectroscopic timeseries data.
- There are no obvious and/or unexpected systematic errors that limit the interpretation of
the data. We have not yet identified a noise floor for JWST timeseries data. As we
average more data together, the precision continues to improve. - Data from different instruments are consistent in the overlap regions.
These finding have profound implications. For example, several pre-launch studies were conducted to predict JWST capabilities for transiting exoplanet science. Among those were studies of the seven terrestrial-size planets orbiting the nearby M-type star, TRAPPIST-1. Three of its planets orbit in the Habitable Zone. Those studies predicted that JWST would be capable of detecting the presence of an atmosphere should one exist and identify the dominant background gas assuming enough transits are observed. Pre-launch predictions were based on models of how we thought the telescope would perform. Now we have ground-truth observations that confirm the models and confirm that we can achieve our science goals with JWST even for these challenging exoplanets.
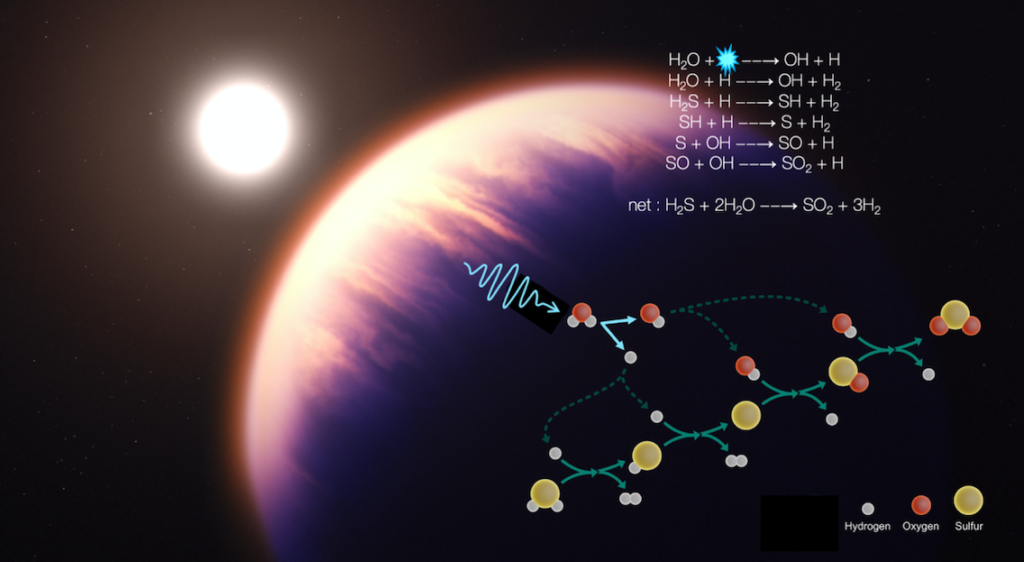
Figure 4. Detailed modeling reveals that SO2 in the WASP-39b atmosphere is produced by photochemistry – chemical reactions catalyzed by high-energy starlight (light blue symbols). Photochemistry is fundamental for life on Earth to thrive, from the production of ozone in the upper atmosphere to photosynthesis in plants and algae to the production of Vitamin D in our skin. (Credit: R. Hurt, JPL)
Looking Forward:
With its unprecedented precision, stability, and infrared wavelength coverage, JWST will catalyze a new epoch of exoplanet exploration characterized by atmospheric studies. Over 70 transiting exoplanets will be observed by JWST in Cycle 1 alone. Over its lifetime, hundreds could be studied providing a new lens on exoplanet diversity and a new survey data for demographic studies. From these data, we gain deep insights into planet formation and evolution processes. We will learn about the nature of the mysterious but common super-earths/mini- neptunes. We will know if terrestrial-size planets orbiting M dwarfs have atmospheres, and we will lay the groundwork for identifying habitable environments and living worlds in the future.
Bibliography
[1] Identification of carbon dioxide in an exoplanet atmosphere, The JWST Transiting Exoplanet Community Early Release Science Team, (2022), arXiv, arXiv:2208.11692
[2] Spectroscopic Time-series Performance of JWST/NIRSpec from Commissioning Observations, Espinoza, N., Úbeda, L., Birkmann, S. M., Ferruit, P., Valenti, J. A., Sing, D. K., Rustamkulov, Z., Regan, M., Kendrew, S., Sabbi, E., Schlawin, E., Beatty, T., Albert, L., Greene, T. P., Nikolov, N., Karakla, D., Keyes, C., Kumari, N., Alves de Oliveira, C., Böker, T., Peña-Guerrero, M., Giardino, G., Manjavacas, E., Proffitt, C., & Rawle, T., (2022), arXiv, arXiv:2211.01459
Astrobiology, Exobiology,