Study Pinpoints Process That Might Have Led To First Organic Molecules
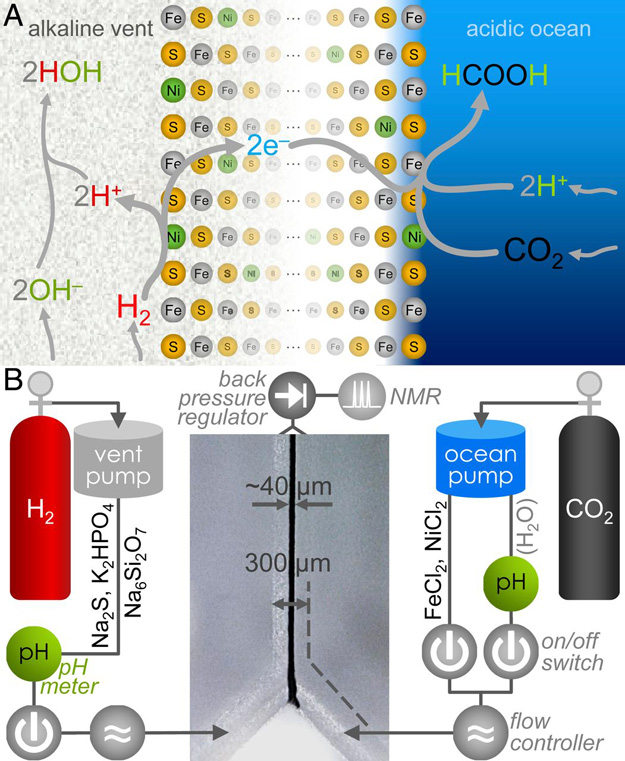
New research led by the American Museum of Natural History and funded by NASA identifies a process that might have been key in producing the first organic molecules on Earth about 4 billion years ago, before the origin of life. The process, which is similar to what might have occurred in some ancient underwater hydrothermal vents, may also have relevance to the search for life elsewhere in the universe. Details of the study are published this week in the journal Proceedings of the National Academy of Sciences.
All life on Earth is built of organic molecules–compounds made of carbon atoms bound to atoms of other elements such as hydrogen, nitrogen and oxygen.
In modern life, most of these organic molecules originate from the reduction of carbon dioxide (CO2) through several “carbon-fixation” pathways (such as photosynthesis in plants). But most of these pathways either require energy from the cell in order to work, or were thought to have evolved relatively late. So how did the first organic molecules arise, before the origin of life?
To tackle this question, Museum Gerstner Scholar Victor Sojo and Reuben Hudson from the College of the Atlantic in Maine devised a novel setup based on microfluidic reactors, tiny self-contained laboratories that allow scientists to study the behavior of fluids–and in this case, gases as well–on the microscale. Previous versions of the reactor attempted to mix bubbles of hydrogen gas and CO2 in liquid but no reduction occurred, possibly because the highly volatile hydrogen gas escaped before it had a chance to react. The solution came in discussions between Sojo and Hudson, who shared a lab bench at the RIKEN Center for Sustainable Resource Science in Saitama, Japan. The final reactor was built in Hudson’s laboratory in Maine.
“Instead of bubbling the gases within the fluids before the reaction, the main innovation of the new reactor is that the fluids are driven by the gases themselves, so there is very little chance for them to escape,” Hudson said.
The researchers used their design to combine hydrogen with CO2 to produce an organic molecule called formic acid (HCOOH). This synthetic process resembles the only known CO2-fixation pathway that does not require a supply of energy overall, called the Wood-Ljungdahl acetyl-CoA pathway. In turn, this process resembles reactions that might have taken place in ancient oceanic hydrothermal vents.
“The consequences extend far beyond our own biosphere,” Sojo said. “Similar hydrothermal systems might exist today elsewhere in the solar system, most noticeably in Enceladus and Europa–moons of Saturn and Jupiter, respectively–and so predictably in other water-rocky worlds throughout the universe.”
“Understanding how carbon dioxide can be reduced under mild geological conditions is important for evaluating the possibility of an origin of life on other worlds, which feeds into understanding how common or rare life may be in the universe,” added Laurie Barge from NASA’s Jet Propulsion Laboratory, an author on the study.
The researchers turned CO2 into organic molecules using relatively mild conditions, which means the findings may also have relevance for environmental chemistry. In the face of the ongoing climate crisis, there is an ongoing search for new methods of CO2 reduction.
“The results of this paper touch on multiple themes: from understanding the origins of metabolism, to the geochemistry that underpins the hydrogen and carbon cycles on Earth, and also to green chemistry applications, where the bio-geo-inspired work can help promote chemical reactions under mild conditions,” added Shawn E. McGlynn, also an author of the study, based at the Tokyo Institute of Technology.
###
Other authors on this study include Ruvan de Graaf and Mari Strandoo Rodin from the College of the Atlantic, Aya Ohno from the RIKEN Center for Sustainable Resource Science in Japan, Nick Lane from University College London, Yoichi M.A. Yamada from RIKEN, Ryuhei Nakamura from RIKEN and Tokyo Institute of Technology, and Dieter Braun from Ludwig-Maximilians University in Munich.
This work was supported in part by NASA’s Maine Space Grant Consortium (SG-19-14 and SG-20-19), the U.S. National Science Foundation (1415189 and 1724300), the Japan Society for the Promotion of Science (FY2016-PE-16047 and FY2016-PE-16721), the National Institutes of Health’s National Institute of General Medical Sciences (P20GM103423), the European Molecular Biology Organization (ALTF- 725 1455-2015), the Institute for Advanced Study in Berlin, and the Gerstner Family Foundation.
CO2 reduction driven by a pH gradient, PNAS
Astrobiology, Astrochemistry