Terrestrial Analogs to Mars: Planetary Decadal Study Community White Paper Solar System Exploration Survey, 2003-2013
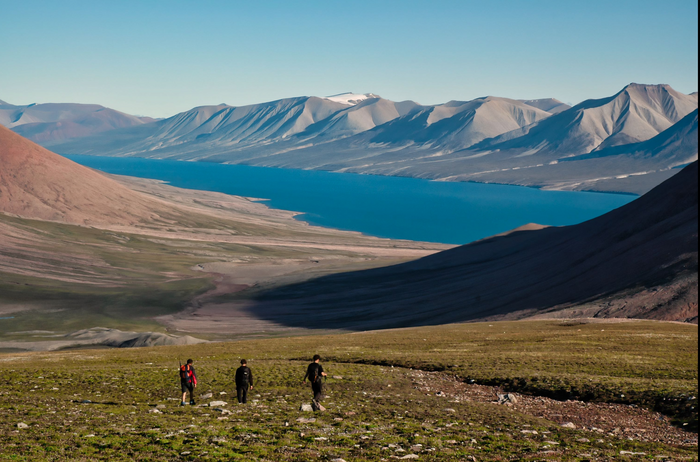
Planetary Decadal Study Community White Paper Solar System Exploration Survey, 2003-2013
SUBJECT AREA: Mars DRAFT DATE: 12/07/01
Terrestrial Analogs to Mars
Executive Summary
CURRENT STATE OF KNOWLEDGE:
It is well recognized that interpretations of Mars must begin with the Earth as a reference. The most successful comparisons have focused on understanding geologic processes on the Earth well enough to extrapolate to Mars’ environment. Several facets of terrestrial analog studies have been pursued and are continuing. These studies include field workshops, process studies at analog field sites, instrument and operations tests, physical modeling, and laboratory measurements.
KEY SCIENCE QUESTIONS:
The key questions can be divided into 1) Science and 2) Technology/operations areas.
1) The overarching scientific goals of the Mars program have been documented by the Mars Exploration Payload Assessment Group. They include: a) Detect life or its remnants, b) Determine present and past climate and its drivers, c) Determine the geologic processes that have created and modified Mars, and d) Prepare for human exploration. Underlying this is the fact that we are entering a new phase of Mars study: The era of global mapping is ending and studies will begin to focus on detailed analysis at the regional to outcrop level. Analog studies will play a crucial role in this transition.
2) Prediction of capabilities and interpretation of data from future instruments is also a critical need. By optimizing the choice of instruments and their operating parameters through tests in analog environments on Earth, we maximize our chances for success. Instruments in need of such study include low-frequency airborne or orbital radar sounders; low-frequency airborne or orbital imaging radar; surface ground penetrating radar and EM sounders; drills; rovers; high resolution stereo imagers from orbit, lander, or microscope; high resolution topography; hyperspectral visible, near-IR, thermal IR; Raman; Mossbauer; SEM/EDAX, Laser-Induced Breakdown Spectroscopy. Accurate georeferencing or geocoding of these data needs to be developed operationally. In addition, Mars surface operations, both robotic and human, need to be developed and tested in analog settings.
RECOMMENDATIONS:
Much Mars analog research has been accomplished and a great deal of activity is currently ongoing. However, more coordination among the many current individual projects could yield additional benefits. Focusing the community on a few field sites will also help build the diverse remote sensing data sets and supporting data needed to interpret their equivalents from Mars. This should particularly benefit the technology and operations development community as new instruments and techniques need testing. Laboratory measurements are another area in which ongoing efforts could be enhanced through appropriate funding.
Coordinated deployment of airborne, spaceborne, field instrumentation, and personnel to several sites to test instruments, technology, and exploration strategies intended for Mars and to provide data for ongoing studies of terrestrial geologic processes relevant to Mars is the most important area for support. The support will be used to leverage current investments by NASA codes S (Space Science), Y (Earth Science), and M (Human Exploration); NSF; US Army CRREL; and international agencies by acquiring additional data sets at important analog sites which are being studied for a variety of reasons. These activities should begin as soon as possible in order to benefit current and upcoming Mars missions.
Places where activities should commence as soon as possible include desert sites in the western US, central Australia, the Sahara Desert in Egypt, and the Dead Sea. High-priority cold regions to be studied include Antarctica as well as sites in Alaska, Canada, and Iceland. Volcanic areas of high priority include Hawaii, the Snake River Plain in Idaho, Kamchatka, Iceland, Mt Pinatubo in the Philippines, and the East Africa Rift in the eastern horn of Africa. Young impact craters such as Meteor Crater, Arizona; Zhamanshin, Kazakhstan; Roter Kamm, Namibia; and several in Australia should also be given priority. These are all areas where significant activities are already underway, so that this program would be enhancing the data collection to improve applications to the study of Mars.
Costs for this part of the program will be modest for small enhancements to ongoing work in easily accessed areas, but high for coordinated multi-aircraft and field campaigns to places like Antarctica. These costs should be shared across agencies and it will require significant cross-agency and international coordination to make the larger projects happen.
Data archives are an important facet of this program. They would collect, document, and make available via the Web existing terrestrial data relevant to Mars studies. These could include various remote sensing and radar sounder data of important sites, laboratory data, and a comprehensive bibliography including actual articles and data files. The existing Planetary Data System could host these archives. A working group is needed to identify data sets and appropriate formats for inclusion.
Field workshops should be continued and expanded to include a wider range of researchers, with guidebooks published on paper as well as Web-based for wider community access. These field workshops could also include operation of field instruments similar to those intended for Mars, modeled after the Marsokhod field tests at Silver Lake (Stoker et al., 2001). The Lunar and Planetary Institute is prepared to assist in workshop organization as it did for the Martian Highlands/Mojave Desert Workshop (Howard et al., 2001).
Laboratory measurements are ongoing, however this panel identified several areas that should be given a high priority. There is a critical need to characterize more fully various Mars analog materials, such as clathrates, palagonites, and rock coatings. Electromagnetic characterization of Martian analogs, such as ices, evaporites, clays and magnetic minerals needs to continue in support of the definition of future subsurface radar sounders.
OTHER
Human exploration.
Mars analog studies and geological field training exercises will be crucial in the human exploration of Mars because of its rich and complex geological history (perhaps even biological history). At analog sites, astronauts will be able to carry out the proper field investigations for correct geological context of the landing site as well as the surrounding environs, conduct precision landings, maximize surface mobility, and also perform intelligent sample acquisition and interpretation.
Education and Public Outreach.
The more accessible sites especially could become “standard” sets for filmed news stories and documentaries. Demonstrations of rovers are always popular- more so in a Mars-like setting. The public can be encouraged to visit some of the sites, yielding an opportunity to describe how the site is like Mars. National Monuments and Parks such as Death Valley, Mojave Desert, and Hawaii Volcanoes may be interested in helping to support this.
REPORT
CURRENT STATE OF KNOWLEDGE
1. Introduction
It is well recognized that interpretations of Mars must begin with the Earth as a reference. The most successful comparisons have focused on understanding geologic processes on the Earth well enough to extrapolate to Mars’ environment.
Several facets of terrestrial analog studies have been pursued and are continuing. These studies include field workshops, process studies at terrestrial analog sites, instrument and operations tests, laboratory measurements, and computer and laboratory modeling. The combination of all these activities allows scientists to constrain the processes operating in specific terrestrial environments and extrapolate how similar processes could affect Mars.
In this section we attempt to summarize some of the past and current activities in these areas to set the stage for a set of Recommendations.
2. Field workshops
Field workshops have been recognized as a very important aspect of comparative planetology training since before the Apollo missions to the Moon. The Apollo astronauts underwent field training under the supervision of planetary and terrestrial geologists in order to better recognize and return lunar rocks of particular importance to our understanding of the geologic processes that have operated on the Moon. At present, astronaut candidate classes receive a week of field geological training in the Rio Grande rift, including geophysical data acquisition. As we continue our exploration of Mars with robotic landers and rovers and prepare for a future that likely will include human exploration, we again find that the terrestrial experience is invaluable in interpreting the remote sensing data being returned by the various Mars missions. Planetary geologists have organized a number of field workshops in order to train each other in the interpretation of Mars mission data. Terrestrial analog sites are identified based on their similarity to specific environments on Mars.
Locations that have been utilized in field workshops and general conferences as terrestrial analogs to Mars include the following:
Mars Feature/Environment | Terrestrial Analog Site | References |
Low viscosity volcanism | Hawaii | Greeley, 1974 |
Snake River, Idaho | Greeley and King, 1977 | |
Wrinkle Ridges | Columbia River Plateau | Watters and Golombek, 1989 |
Outflow Channels | Channeled Scablands | Baker and Nummedal, 1978 |
Golombek et al., 1995 | ||
Valley Networks | Colorado Plateau | Howard et al., 1988 |
Devon Island | Lee and Rice, 1999 | |
Gullies | Devon Island | Lee et al. 2001 |
Greenland | Costard et al 2001 | |
Paleo-lakes | Bonneville, Utah | Rice, 1992 |
Devon Island | Lee et al., 1998 | |
Aeolian Features | Southern California | Greeley, et al., 1978 |
Southwest Egypt | El-Baz and Maxwell, 1982 | |
Glacial/Permafrost Features | Alaska | Kargel et al., 1993 |
Devon Island | Lee et al., 1998, Lee 2000 | |
Canada | Grasby et al., 2001 | |
Mars Polar Science | Iceland | Clifford et al., 2000 |
Volcano-Ice Interactions | Iceland | Chapman et al., 2000 |
Spectral Analogs | Hawaii | Evans and Weitz, 1999 |
Fluvial, Aeolian Features | Death Valley, Mojave | Howard et al., 2001 |
Banded Iron Formations | Canada | Allen et al., 2001 |
Field workshops have been a common addition to the Planetary Geology Mappers meetings supported by NASA. Examples of some of the field trips that have been held in association with these meetings are shoreline analogs at the Great Salt Lake and Lake Bonneville, Utah (1992), glacial features in northwestern New York (1999), and volcanic features near Flagstaff, AZ, (1998) and Albuquerque, NM (2001). Several workshops have also been run by the University of Hawaii for graduate students, post-docs, and junior faculty. The workshops have focused on comparisons between Kilauea and Mauna Loa volcanoes and Mars. Remote sensing data from visible, near infrared, thermal infrared, and radar sensors over lava flows, cones, and calderas are used to help interpret Viking, MGS, and Earth-based data for Mars. A comprehensive field guide and set of remote sensing examples are provided to the workshop participants. The next workshop is planned for summer 2003. Discussions are underway for a possible summer workshop in the Canadian High Arctic in the future which will include opportunities for field visits to several sites of interest (P. Lee and W. Pollard, personal communication).
3. Process studies
Study of potential geologic processes operating on Mars through study of similar processes at analog field sites on Earth makes the results easier to extrapolate and less site-specific. Processes of interest include: Processes that form and modify surfaces in arid and polar regions (e.g. aeolian, flood, periglacial, mass-wasting, glacial, rock weathering, lacustrine/marine, evaporites), ice and glaciers, volcanic processes (e.g. explosive, effusive, lahars, hydrothermal), tectonic (faulting, folding), magmatic/rock-forming processes, impacts, and radiation. A number of sites have been studied or are currently being studied for their application to understanding geologic processes on Mars. The following list attempts to summarize sites at which significant work has been done or is ongoing. Future work is proposed in the Recommendations Section.
3.a. Surficial processes (aeolian, floods, mass-wasting, rock weathering, lacustrine/marine, evaporite)
Mojave/Death Valley:
The Mojave Desert/Death Valley region of southeastern California is currently a dry environment but climatic conditions in the past favored wetter conditions. Many examples of dry lake beds (playas) are found in this region and serve as excellent terrestrial analogs to martian basins proposed to contain paleolake deposits and evaporites (Baldridge et al., 2001). As the evidence continues to mount that Mars has experienced warmer and wetter periods throughout its history, areas such as the Mojave Desert and Death Valley become increasingly important as terrestrial analog sites. In addition to the playa deposits, many other geologic processes have affected this region, including aeolian (sand dunes, sand sheets, and dust devil activity ), tectonism and volcanism which also have implications for the development of the martian highlands. The importance of the southeastern California desert region to understanding Mars is demonstrated by the early field guide developed for this region (Greeley et al., 1978) and the October 2001 workshop on its applicability as a terrestrial analog to the martian highlands (Howard et al., 2001).
Arizona:
This past summer, the MATADOR team put together a field expedition to Eloy, Arizona, to pursue dust devils with about a dozen instruments. MATADOR is an atmospheric dust payload developed in response to an AO released two years ago by the HEDS organization for the planned 2003 Mars Lander. The lander has since been cancelled, and the small amount of funding was used to explore measurement strategies in this Earth analog location, chosen for the frequent appearance of vortices. MATADOR is a combination of meteorological instruments (wind, temperature, pressure), dust impact devices (triboelectric and momentum), electrostatic sensors (total E-field and radio noise, or spherics), a camera, an oxidant sensor, and lidar.
Colorado River Plateau:
The small valley network systems seen throughout the martian highlands and on the flanks of some volcanoes have been argued to result from rainfall and surficial runoff and by groundwater sapping. Many of the morphologic features of the valley networks are characteristic of sapping valleys seen on the Earth. One example of sapping valleys on Earth that is commonly used as a terrestrial analog for the valley networks of Mars are the channels found along the Colorado River Plateau in the southwestern United States. The sapping valleys are particularly well developed in southeastern Utah (Laity and Pieri, 1980), where headwater retreat and hanging valleys characterize the morphology. The debate between a rainfall versus sapping origin for the martian valley networks continues as higher resolution imagery becomes available, so such terrestrial analogs are expected to continue to play a role in the interpretation of the martian data.
Channeled Scablands of the Northwestern United States:
The huge outflow channels found on Mars have a terrestrial analog in the Channeled Scablands of the northwestern United States. This area, although smaller than the martian channels, displays the scour marks, “tadpole”-shaped islands, and other features characteristic of erosion by catastrophic floods. The Channeled Scablands were created by several episodes of catastrophic flooding associated with failures of ice dams during the Quaternary period. The last major episode of flooding extended from about 18,000 to 13,000 years ago when floodwaters from Lake Missoula spilled out over eastern Washington and down the Columbia River basin to the Pacific Ocean. Baker and Nummedal (1978) describe the similarities between the Channeled Scablands and the Martian outflow channels. The Channeled Scablands have been used as a terrestrial analog for outflow channels on many field workshops, including a pre-landing workshop to characterize the Mars Pathfinder landing site (Golombek et al., 1995).
Central Australia:
Next to Antarctica, Australia is the driest continent on Earth. Aridity began ~5 million years ago, but a number of climatic oscillations have occurred through the Quaternary. This climatic evolution has created many landforms that are unique to Australia, including low relief deserts with extensive duricrust plains, stony deserts, a continental-scale whorl of longitudinal dunes, drainage-aligned playas and clay pans, and a network of disorganized internal drainage systems. Although the usefulness of any of these features as potential martian analogs is obvious, little work has been done in this regards. Bourke and Zimbelman (2001) present a compelling case that rivers in central Australia share morphologic characteristics with many martian valley networks. This implies that many fluvial deposits on Mars may have resulted from unconfined flow, and thus should be easier to identify in orbital images.
Because of the great age of its basement rocks, central Australia contains numerous impact structures that have become type examples for understanding cratering as a geologic process. Gosses Bluff, for example established the importance of shatter cones as one of the features diagnostic of impact cratering (Milton et al., 1972).
It is also worth noting that Earth’s earliest biologic record is recorded in a variety of outcrops located in central Australia. Notable examples include microfossils found in the Bitter Springs formation and stromatolites common in the Warrawoona group.
Sahara Desert:
The Eastern Sahara, including Egypt’s Western Desert and neighboring parts of Sudan and Libya, is the site of late Quaternary river networks buried by several meters of sand and recently revealed by Shuttle Imaging Radar (Schaber et al., 1986, 1997; McCauley et al., 1986; Abdelsalam and Stern, 1996; Stern and Abdelsalam, 1996). In February 2000, at a test site located in the Southern Egyptian Desert, at the Bir Safsaf oasis, these paleo-channels were mapped by French scientists using 500-900 MHz ground penetrating radar. Additional tests are planned for the coming years (see Recommendations Section).
Tunisia:
The Playa deposits of Chott el Jerid and el Gharsa in Tunisia have been studied as a Mars lacustrine analog. The area is located on the southern front of the Atlas thrust and fold belt. Chott el Jerid and Chott el Gharsa are environments undergoing dramatic changes at different scales and show a combination of aeolian, fluvial and lacustrine processes (Ori et al., 2001). This combination of geological environments is very similar to what has been observed on the surface of Mars. The chotts are underlain by a thick accumulation of Cretaceous and Tertiary (Mio-Pliocene) sediments that also contain gypsum-rich ancient marine evaporites. The lacustrine evaporite formation is an ongoing process and a large quantity of new evaporite minerals are formed after major flooding events. This pulsating hydrological cycle with episodic discharge and basin filling could be a good comparison with the martian lacustrine system. Detailed analysis of the chott area is underway using multispectral and radar data, supported by fieldwork.
Dead Sea:
Evaporites have been collected recently for study of microbial populations and fossilization in this environment (Morris et al., 2000; Wentworth and Morris, 2001).
3.b. Polar processes (aeolian, permafrost, periglacial, mass-wasting, glacial):
Antarctic Dry Valleys:
The ice free desert regions of Antarctica are no doubt the best Mars analogs in terms of mean annual temperature, aridity, winds, and remoteness. These regions also contain relevant martian analogs such as ancient lake deposits, deltas, sand dunes, cinder cones, and periglacial processes and landforms. Glacial landforms have been postulated for Mars and these regions obviously contain glacial landforms. The field investigation of Antarctic microbial life habitats (i.e., endoliths, crypto-endoliths, chasmaliths) would also provide useful training for Mars missions.
Antarctic sub-ice lakes:
With the emergence of a new field of exobiology to study plausible extraterrestrial life, there has been an increasing interest in Antarctic sub-ice lakes. About 77 lakes have been identified in Antarctica under the kilometers-thick ice cover from airborne radio-echo-sounding data of the early 1970’s (Oswald and Robin, 1973; Siegert et al., 1996). Radio altimeter data of the ice above Lake Vostok by the European satellite ERS-1 in 1993 coincided with the revised interpretation of seismic data obtained by Russian scientists in 1964 (Kapitsa et al., 1996). These data are complemented by the more recent radar imaging of the ice surface by the Canadian Radarsat satellite (September-October 1997) and the aerial geophysical survey of Lake Vostok in 2000-2001 by the University of Texas Institute for Geophysics (UTIG) including ice-penetrating radar, laser altimetry, gravity, and magnetics (Holt, 2001).
A modeling study by Duxbury et al. (2001) concluded that Lake Vostok could have survived the Antarctic glaciation without freezing completely, supporting an alternative to a basal geothermal melting origin. The numerical experiments of Duxbury et al. (2001), in combination with the recent Mars Global Surveyor Mars Orbiter Laser Altimeter (MOLA) data, also support the existence of subpolar water on Mars, even though the Martian ice caps are not thick enough to generate geothermal melting. Their model for the lake’s origin relaxes the condition on the thickness of Mars’s polar caps, i.e. potential subglacial lakes can exist on Mars under much thinner ice than it was thought before. As with Lake Vostok on Earth, the Duxbury et al. (2001) model has interesting implications for the search for life (either extant or extinct) on Mars: If there are lakes under the martian polar caps, they might be the oldest preserved aquifers on Mars, and perhaps the most accessible sources of liquid water and extant or extinct life.
Antarctic ice sheets:
Reliable detection and characterization of any sub-ice lakes or smaller scale water systems beneath the Mars polar caps will depend on a foundation of analogous interpretations for the Antarctic ice sheet. Most importantly, identification of any sub-ice water systems on Mars using radar sounding techniques will require detailed knowledge of the physical properties of the intervening ice. This is because attenuation of radar waves is a function of the temperature as well as the impurity content of the overlying ice column. New orbital observations of the surface elevation of Mars’ northern polar cap (Zuber et al., 1998) have allowed detailed thermo-mechanical modeling of its internal properties (e.g., Larsen and Dahl-Jensen, 2000). This has set the stage for calculations required to interpret future orbital radar sounding data collected over the ice caps. The process of interpreting sub-ice water from airborne radar sounding data is well established for the Antarctic ice sheet (Oswald and Robin, 1973, Bentley et al., 1998). Similarly, new approaches are beginning to be used in attempts to more fully characterize sub-ice water systems in both West and East Antarctica using phase-coherent airborne radar sounding (e.g. Peters et al., 2001). Phase coherent radar surveys have recently been completed by UTIG over Lake Vostok in East Antarctica and Ice Stream D in West Antarctica.
A number of expeditions have been carried out to melt or otherwise penetrate ice sheets, a precursor to a Mars polar subsurface expedition. A group just returned from the Svalbard glacier off the coast of Norway after penetrating over 20 meters with a prototype of a Mars cryobot (Anderson et al., 2001).
Alaska/Canada:
A number of regions, environments, and landforms in Alaska and Canada have potential for use in Mars analog research. A large proportion of the region is at present within the discontinuous and continuous permafrost zones, offering many opportunities for understanding the influence of permafrost processes on geological materials and features. The modern periglacial environment in the Canadian High Arctic is extensive, and surface exposure of bedrock and associated weathered materials within this polar desert is very good. Much of the region is far more accessible than similar terrain found in Antarctica. The entire landmass of Alaska and Canada has been repeatedly subjected to glacial and cold-climate conditions during the Quaternary, providing researchers today with a natural laboratory for understanding surface processes and features related to ice sheet properties and dynamics, glacio-isostacy, proglacial lakes and associated drainage systems. In addition, numerous impact features have been recognized, both on the Canadian Shield, and on geological units of the Phanerozoic (World Crater Inventory; Grieve et al., 1988). A field workshop was held in Alaska in 1993 to show the similarities of terrestrial eskers, pingos, polygonal terrain, and maars to features seen on Mars (Kargel et al., 1993). Future workshops in this area are planned to address the issues of impacts into ice-rich environments. The exceptionally well-exposed and preserved Haughton impact structure on Devon Island has been the site of rover mission simulations and simulation activities regarding human crews. The presence of both impact processes and periglacial processes makes it an especially good analog for continuing work.
Devon Island:
Devon Island is located in the Canadian High Arctic Archipelago in Nunavut Territory. It bears one of the highest-latitude terrestrial impact structures known on Earth, Haughton Crater. Located at 75°26’ N, 89°51’ W, it is the only impact structure known to be set in a polar desert, albeit one that is less extreme than Mars. Ongoing research conducted since 1997 under the auspices of the NASA Haughton-Mars Project (HMP) has revealed a wide variety of possible parallels between features on Devon Island and possible counterparts on Mars (e.g., Lee 1997, Lee et al. 1998, Lee 2000). At Haughton Crater, analog studies may focus on impact processes, their broad range of resulting features, and the post-impact modification of an impact structure under a climate that has been predominantly cold and relatively dry since the structure’s formation. Studies of how an impact record (including intracrater paleolacustrine features) may be preserved and exposed under the present polar desert setting is of unique value, in particular for helping plan the exploration of similar sites on Mars (e.g., Lee 1993, Glass and Lee 2001, Osinski et al. 2001). More generally across Devon Island (including at Haughton), a wide variety of periglacial, niveal, glacial, fluvial, eolian, lacustrine, mass-wasting, and polar weathering features and processes can be investigated. Analog studies on Devon Island conducted by the HMP team are beginning to point to the possible key role played by cold climate niveal and glacial processes, including meltwater processes, in the formation of a variety of classically-called “fluvial” features on Mars (Lee and Rice 1999, Lee et al. 1999, Lee 2000, Lee et al. 2001). Microbiology studies on Devon Island also offer the opportunity to investigate the possibilities, adaptations and limits of life in the relatively extreme environment of the High Arctic, in particular in the context of an impact structure (Cockell and Lee 2001; Cockell et al. 2001; www.marsonearth.org).
Iceland Floods:
The most voluminous and catastrophic floods known to occur on Earth in historic times are the Icelandic jökulhlaups, the largest of which can have peak discharges on the order of 106 m3/s and depths of 60-70 m. Jökulhlaups are caused by the abrupt drainage of ice dammed lakes or subglacial volcanic eruptions. The probable causes of Martian floods are massive releases of subsurface water/ice due to possible subsurface volcanic activity, mechanisms very similar to the release of jökulhlaups in Iceland. Another important aspect of jökulhlaups is that channel systems begin as point sources, at glacier termini or lacustrine breakout points, and flow is then directed along one or two primary incised channels until it emerges as unconfined outwash. This is analogous to Martian outflow channels, which also begin at point sources (chaotic terrain and box canyons), and then flow unconfined into a basin region. Erosional landforms associated with jökulhlaups include streamlined hills, extensive washed/ stripped lava flows, and cataracts. Depositional landforms consist of pendant bars, boulder bars, imbricated boulder trains, transverse ribs, and giant current ripples. Many of these landforms are also found on Mars (Rice, 2001; Rice et al., 2000).
3.c. Volcanic
Hawaii:
Hawaii has been used extensively for (a) developing ideas on the eruption and emplacement of lava flows (e.g., Macdonald, 1953; Peterson and Tilling, 1980; Hon et al., 1994); (b) analysis of the structure of basaltic volcanoes on Earth and Mars, including rift zones, calderas, and regional slopes (e.g., Rhodes and Lockwood, 1995); (c) weathering processes (Farr and Adams, 1984); (d) trafficability studies for Mars rovers; (e) education and public outreach. Hawaii is especially useful for the understanding of volcanic processes because of the near-continuous activity at Kilauea Volcano. No other site on the planet provides this mix of active volcanic processes and easy access. In addition to Kilauea, Mauna Loa (last erupted in 1984) is the largest volcano on Earth, providing a structural and volcanological analog to the even larger shield volcanoes on Mars. Hualalai and Mauna Kea provide excellent examples of more evolved eruptive products, which may have some relation to the “andesites” reported from Mars. Coordination with the USGS Hawaii Volcano Observatory would greatly increase the science value of future workshops in this area. Coordination with the US National Park Service and the native Hawaiian groups is also required for expanded studies.
Snake River Plain:
The Snake River Plain in Idaho consists of a variety of low-viscosity basaltic lava flows emplaced primarily by fissure eruptions. These extensive volcanic plains serve as a good terrestrial analog to the volcanic plains of Mars, including the ridged plains and lava flows extending outward from the Tharsis and Elysium volcanoes. The use of the Snake River Plain as a terrestrial analog for extensive sheet lavas not only on Mars but also on Venus and the Moon has resulted in their being the destination for several workshops (Greeley and King, 1977), including a Planetary Geologic Mappers meeting in 1994. As high resolution imagery continues to be collected on the volcanic plains of Mars, this region will continue to serve as an excellent terrestrial analog for the study of lava channels, aa versus pahoehoe flows, and fissure vents which are associated with such flows.
East African Rift:
Tectonic and volcanic processes have been studied extensively in the East Africa Rift. Recently French scientists analyzed the composition of the basalts and weathering products and found strong similarity to Mars’ composition. In particular, many iron-bearing phases were found, including ferromagnetic materials (Paillou et al., 2001).
Iceland:
Iceland is a land of fire and ice, with the inevitable catastrophic floods when mixed. The largest basaltic eruption recorded by humans was the 1783-1784 Laki eruption (e.g., Thordarson and Self, 1993). This immense lava flow produced a lava morphology unlike any commonly seen in Hawaii, but apparently very similar to the flood lavas on Mars (Keszthelyi et al., 2000). Iceland is the only place on Earth that eyewitness descriptions of lava flows approaching the scale of flood basalt eruptions can be examined. There are a number of other pre-historic Icelandic lava flows that are larger in size, and similar in morphology to the Laki flows (Self et al., 1998). Rootless cones, formed by the explosive interaction of wet sediments and liquid lava, were first recognized in Iceland because they are very common and easy to observe there (Thorarinsson, 1953). Martian examples of rootless cones are of great interest because they are proof of water/ice within the shallow subsurface (e.g., Lanagan et al., 2001). Iceland is also the home of recent sub-glacial eruptions that produce catastrophic floods (the last major example was in 1996) as well as tuyas, km-scale mesa-like landforms (Chapman, in press). Iceland provides by far the most accessible location to study the interaction between volcanic, glacial, and fluvial processes (McEwen et al., 2001).
Peru:
The objective of these studies are to improve understanding of the emplacement of thick, evolved lava flows by studying those at Sabancaya volcano in Southern Peru (Bulmer at al., 1999). Inferences have been made on the rheology and flow regime of the lava flows based on the dimensions, topography, morphology, petrology and geochemistry of the flows (Bulmer and Gregg, 2001; Gregg et al., 2001). In addition, an examination has been made of the extent to which surface structure and roughness of different lava flow surfaces can be used as a diagnostic tool for accurate geologic interpretation of the emplacement styles and lava compositions from remotely sensed data on Earth and the terrestrial planets (Bulmer et al, 2001; Shepard et al., 2001).
Mt Pinatubo:
Extensive data on the erosion of the lahars from the recent eruption have been collected by the University of Hawaii and the Philippine Volcano Observatory. Lahar movement on Pinatubo over the ten years since its most recent eruption has been mapped and quantified by Evans (2001) using detailed astronaut-acquired photographs.
Queensland, Australia
A workshop held in 1996 in Queensland, Australia, sponsored by AGU was convened to examine long lava flows. Young (<2 ma) basalt flows are well preserved there due to the climate, and furthermore represent some of the longest subaerial flows on Earth. A single lava tube, emanating from Undara volcano, can be traced for ~160 km. The excellent preservation and great lengths of these flows make them ideal analogs for long martian lava flows.
3.d. Tectonic
Columbia Plateau:
The Columbia Plateau in the northwestern United States has been used as a terrestrial analog site for the study of wrinkle ridges (Watters and Golombek, 1989; Mege and Reidel, 2001). Wrinkle ridges are seen not only on the lunar mare but also on the venusian plains and the martian ridged plains. They are believed to form from compressive stresses. An excellent terrestrial example of a wrinkle ridge is the Yakima Fold Belt, found in the Columbia River Plateau of central Washington. This feature is a series of anticlinal ridges and synclinal valleys composed of basalt flows from the Columbia River Basalt Group with interbedded sediments. The area was folded and faulted under north-south directed compression, perhaps associated with the formation of the Cascade volcanic range to the west and the Rocky Mountains to the east, or with the Yellowstone hotspot in a manner possibly analogous to wrinkle ridge formation associated with Tharsis on Mars (Mege and Ernst, 2001). The Yakima Fold Belt holds great promise for providing better information about the formation and characteristics of the wrinkle ridges seen in the ridged plains and intercrater plains regions of Mars.
3.e. Impact
Impact craters at a variety of sites around the Earth have been studied to learn more about the impact process itself as well as the processes that degrade the original morphology of craters. Sites that have been studied include young craters in arid regions (e.g. Meteor Crater, Arizona; Gosses Bluff and Henbury craters, Australia (Milton, 1968)); and in cold areas (e.g. Haughton, Devon Island; Zhamanshin, Kazakhstan; Popigai, Siberia; Elgygytgyn, Siberia).
Impacts into volatile-rich regions:
There is increasing evidence that the near-surface substrate of Mars contains large quantities of ice into which the vast numbers of impact craters have excavated. The characteristics of impacts into ice-rich or water-rich targets are believed to be quite different from impacts into drier materials. Thus, there is increasing interest to identify and characterize terrestrial impact structures which formed in ice-rich or water-rich environments as well as impacts into targets containing evaporite platform sediments, such as carbonates (Agrinier et al., 2001). Impact structures such as Haughton on Devon Island (Glass and Lee, 2001), Popigai and other impacts in northern Russia (Masaitis, 1976), and a number of recently identified impact craters in Scandinavia are undergoing renewed study as terrestrial analogs for impacts on Mars.
Impact-induced hydrothermal activity:
This may have important biological implications as hydrothermal systems in general may have provided suitable habitats where life may have originated/evolved on Earth and other planets such as Mars. For example, recent work on Devon Island has shown that impact-induced hydrothermal activity occurred following formation of the Haughton impact structure, generating a transient, warm, wet oasis (Osinski et al., 2001).
4. Instrument and technology tests
4.a. Remote Sensing Tests
Geologic Remote Sensing Field Experiment:
The Geologic Remote Sensing Field Experiment (GRSFE) consisted of coordinated airborne remote sensing and field observations over geological targets in the Mojave Desert, California, and the Lunar Lake Volcanic Field, Nevada in 1989. GRSFE was funded by NASA’s Planetary Geology and Geophysics Program, the Geology Program, the Planetary Data System, the Pilot Land Data System, the Mars Observer Project, and the Magellan Project. The GRSFE archive is maintained and updated by the Planetary Data System Geosciences Node at Washington University. Release of the GRSFE archive on a set of CD-ROMs provided a well documented data set for research and teaching. The primary GRSFE objectives were: (a) to acquire airborne remote sensing and field data in a coordinated campaign for key geological targets, e.g., alluvial fans, dunes, lava flows, volcanoes, etc.; (b) to reduce and document the data and deliver the archive to the Planetary Data System (PDS) and the Pilot Land Data System (PLDS); (c) to take advantage of the multisensor approach, allowing cross-comparison of results acquired with different wavelengths; (d) to use results to test quantitative models for the extraction of surface property information from remote sensing data for Earth, Moon, Mars, and Venus; and (e) to use data in prototype terrestrial studies focused on the nature and ages of geological features and implications for regional climatic and tectonic histories. (Arvidson et al., 1993).
Volcanology Data Sets:
Similar to GRSFE, several sets of CDs were compiled with remote sensing, field, and laboratory data for the volcanoes of Kilauea, Mauna Loa, and Kamchatka. Also, since the early 1990’s, measurements have been acquired of the sub-meter topography of Surtsey, the small volcanic islet off the coast of Iceland that formed between 1963-67. Scanning airborne laser altimetry, aerial photos, and IKONOS images have been used. In 1991 the NASA DC-8 collected AIRSAR and AVIRIS data for the island.
ASTER:
The capabilities of the Advanced Spaceborne Thermal Emission Radiometer (ASTER) instrument on the Earth Observing System platform Terra, for lithologic mapping based on spectral unmixing, thermal and slope models is being tested in the Mojave Desert (Galford et al., 2001). Familiarity with the types of data collected by ASTER will help the Mars community as we begin to analyze upcoming data returns from TES and THEMIS. Methods for analyzing ASTER data will be similar to THEMIS analysis methods and ASTER analysis provides an understanding of the strengths, weaknesses and various uses that may be possible for the THEMIS data. These tests are also examining the ability of investigators to “scale up” from lander images to a regional understanding by integrating orbital remote sensing with the landed data. An airborne instrument, MASTER, is also available for rmultispecrtarl visible, near infrared, and thermal infrared data collection.
Hyperspectral:
Guinness et al. (2001) are using Advanced Visible and InfraRed Imaging Spectrometer (AVIRIS) data, to identify and map alteration units at the summit of Mauna Kea. The intent is to understand the processes generating the alteration. In addition to the remote data sets, they are using field work and reflectance measurements of field samples to calibrate the remote spectra and to test the derived maps.
Mass movement monitoring:
The University of Maryland UMBC, USGS, University of Durham, University of Leeds, CNR-IRPI, and University of Ferrara are studying the application of RADARSAT and ERS data under NASA Code Y to investigate potential precursory phenomena of landslides. The goal is to better integrate radar interferograms, field measurements, and ancillary remote sensing at four selected landslides to obtain “calibrated” interferograms, which will provide useful geologic and geophysical information to the landslide monitoring community (Bulmer et al., in prep; Petley et al., 2001). The results from this project have relevance to the design of techniques and methodologies to study landslides on Mars.
Exploration Strategies and Operations:
A team of scientists and engineers from the NASA ARC, NASA JSC, University of Tennessee, Arizona State University, and the University of California at Berkeley have been testing operations strategies for scientific exploration of Mars on Devon Island. Target sites are selected prior to field deployment based on analysis of orbital images. Sites are then prioritized and further investigated using simulated autonomous aerial platforms and rovers, followed by more detailed simulated telerobotic investigation by field team members. The highest priority sites are then explored in simulated human EVA traverses, with significant interaction between the field team and the simulated Science Backroom at ARC.
4.b. Microtopography of desert surfaces
A helicopter-borne, fixed-baseline, dual-camera system for close-range stereophotography has been used in the southwest U.S. to image volcanic, alluvial fan, and playa surfaces. The resulting close-range stereo photographs and microtopographic data can provide input to geomorphic and volcanological studies and radar scattering models for the Earth, Venus, and Mars. (Farr, 1992; Wall et al., 1991)
4.c. Antarctica and the Arctic
The University of Texas Institute for Geophysics (UTIG) is currently funded by NSF to develop airborne radar sounding techniques in Antarctica using a new testbed radar, funded in part by NASA. This is a chirped, coherent system that operates at a center frequency of 60 MHz, has 8 kW of peak power with variable PRF, pulse length and chirp bandwidth. Multiple acquisition systems provide different, simultaneous gain, detector and stacking options. Portions of this radar were developed by JPL in conjunction with the University of Kansas (KU) as a prototype for the Europa Radar Sounder. The remainder was developed by UTIG and the Technical University of Denmark (TUD) and has been used in Antarctica since the early 1970’s (TUD) and 1994 (UTIG). The JPL/KU portion was operationally tested in Antarctica in 2000, and has since been integrated with the other components. The entire system is being operated in Antarctica during the 2001/02 austral summer by UTIG over both East and West Antarctic ice sheet targets. This testbed is being used to evaluate techniques for improved penetration, resolution, and interface characterization, and could form the basis for a new radar design for performing large-scale surveys in polar regions or adaptive surveys of specific targets. It is capable of penetrating over 4000 m of the more absorptive West Antarctic ice.
Dry Valleys:
The US Army Cold Regions Research and Engineering Laboratory (USACRREL) and the Univ. of New Hampshire (UNH), in a joint NSF proposal, visited the Dry valleys in 1998 and 1999. The intent was a pilot study of the ability of ground Penetrating Radar (GPR) to penetrate the glacial deposits there and reveal stratigraphy. The possible Martian analog is that the permafrost is very cold (-18 to -23 C), possibly volcanic, and variable ice content but not ice-rich. GPR frequencies of 50, 100 and 400 MHz showed 35-40 m penetration leading to the conclusion that electromagnetic loss processes are not significant. Most importantly, the presence of volcanic minerals was not an impediment, although the local rock and soil may not necessarily contain magnetic minerals.
UTIG has operated its new testbed radar in the Dry Valleys in order to evaluate techniques for the detection of shallow subsurface water and ice. Targets included permafrost in Taylor Valley (including the USACRREL/UNH GPR sites described above), Beacon Valley (ice body beneath rock glacier), subglacial water below Taylor Glacier, and the grounding line of Ferrar Glacier. Wilson Piedmont Glacier, at the foot of the Dry Valleys, is also part of the regional targets.
West Antarctic ice cap:
USACRREL and UMaine are collaborating on a proposal, funded by NSF, to determine historical accumulation rates and chemical climate records in West Antarctica. CRREL is acquiring high resolution radar stratigraphy using a very small antenna unit (400-MHz) and achieving penetration of more than 100 m in the dry snow and firn, which is about 10X greater than previously achieved by any other researcher at this frequency. The penetration is achieved by high rates of signal trace stacking; the output power is only 1.2 Watts peak, and milliwatts average.
High-resolution airborne radar studies of the ice sheet along the ice divide between the Ross Sea and the Amundsen Sea embayments have been accomplished by UTIG using an 8 kW, short pulse (60 ns) radar sounder operating at 60 MHz (Morse et al., 2001). This reveals internal layers whose thickness variations can be attributed to climatic changes (e.g., Morse et al., 1998) and complements previous large-scale West Antarctic regional surveys by UTIG of surface elevation, ice thickness, magnetics and gravity (Blankenship et al., 1993, 2001; Behrendt et al., 1994, 2001; Holt, 2001). During the 2001/02 season, UTIG is operating its new testbed radar in West Antarctica in order to evaluate techniques for improved layer resolution, characterization of ice stream basal properties, and penetration of the deepest parts of the West Antarctic ice cap (i.e., the Bentley Subglacial Trench).
Devon Island:
A number of studies have been conducted or are underway on Devon Island under the auspices of the
NASA Haughton-Mars Project (HMP) to help develop some of the technologies, strategies and human factors experience needed to help plan the future exploration of Mars by robots and humans.
In support of geological studies specifically, drilling was conducted in 1998 in collaboration with the U.S. Army CRREL to sample permafrosted intracrater paleolakebeds at Haughton Crater without using
any liquid cooling or lubrication of the coring augers and drill bit. The collected core samples are
curated at NASA Ames Research Center and are currently under study for paleoclimatic content.
Ground-penetrating radar surveys were also conducted to study the distribution of ground-ice in
impact-produced materials (breccia formation) and other potential Mars analog features (ice-cored
mounds) (Foessel and Lee, 2001).
5. Laboratory measurements and modeling
5.a. Spectral analogs
Wyatt et al (2001) and Hamilton et al (2001) compared TES spectra (and mineral compositions via a deconvolution technique) of the martian surface with terrestrial basalts and andesites, whose spectra were measured in the lab. This gave an interesting look at how well these rock types could actually be distinguished by seeing if rocks of known composition could be adequately distinguished.
A 3-year project funded by NASA’s Astrobiology program has begun to determine limits of detection (i.e., spatial and spectral resolutions needed) for aqueously-derived minerals in the thermal and near infrared using terrestrial analog sites. So far evaporite basins in Death Valley have been investigated using sensors MASTER (Modis/Aster airborne simulator), ASTER (Advanced Spaceborne Thermal Emission Radiometer on Terra), and AVIRIS (Airborne Visible/Infrared Imaging Spectrometer) (Moersch et al., 2001). In order to make this work more relevant as a terrestrial analog study, plans are to expand to at least one or two more evaporite basins including Salar de Atacama in Chile, the salt pans of Australia, and the Chott el Jerid in Tunisia (in cooperation with G. Ori and G. Komatsu).
5.b. Physical properties of analogs (thermal, electrical, magnetic)
Ices:
Water ice, CO2 ice, and CO2 clathrate hydrate are the principal ices expected to be present in the polar ice caps of Mars and in Martian soil. Laboratory experiments are now giving us well resolved information on the strength of these ices under ductile flow conditions, such as during the slow spreading of an ice sheet under the influence of gravitational forces. Recent results (Durham et al., 2000, 1999a, b) have shown that the three pure phases have very distinct rheologies. The clathrate hydrate is extremely strong and can be considered almost undeformable in comparison with other ices. CO2 in contrast is exceedingly weak and will dominate the behavior of icy mixtures of which it is a component. The strength of water ice is intermediate to that of the other two ices.
Electromagnetic properties:
Several radar experiments are in the planning stages to map the Martian subsurface down to several kilometers, searching for subsurface liquid water reservoirs, all based on the penetration property of radio frequency waves in arid soils. The penetration depth of low frequency radar is mainly related to the electromagnetic properties of the medium, emphasizing the importance of investigations of the electrical and magnetic properties of possible Martian surface and subsurface materials. One approach is to use measurements of the electric permittivity and magnetic permeability of laboratory analogs to then construct a dielectric profile representative of the Martian subsurface (Heggy et al., 2001). Laboratory experiments have also been conducted by Williams and Greeley (2001a,b) to quantify the ability of X-band through P-band radars to penetrate sand and martian analog dust material. These laboratory experiments have been conducted and compared to field measurements and radar images to discuss near-surface penetration abilities of potential imaging radar missions to Mars.
5.c. Studies of Martian meteorites
Current investigations of Martian meteorites utilize a wide range of available analytical techniques including electron microprobe, SEM, TEM, SIMS, ICPMS, XRF, XRD, Raman and Mössbauer spectroscopy, and controlled crystallization experiments. The general goal of most of these studies is to measure compositions of the whole rock, major, minor, and trace elements and isotopes, both stable and radiogenic, all with the aim of understanding the conditions on Mars under which these rocks formed and what processes, if any, were involved in their later alteration. With the new focus in NASA on “Follow the water”, several studies have focused on the involvement of water during formation (e.g. McSween et al, 2001; Dann et al, 2001, Lentz et al, 2001) as well as alteration (e.g. Gooding et al, 1991; Swindle et al, 1997) of the martian meteorites, and what this may imply for the recent water budget, and current water location, for Mars (e.g. Leshin, 2000; Wadhwa, 2001). There have also been a few studies using terrestrial analogs for the martian meteorites. The nakhlites were compared with Theo’s Flow, an archean differentiated lava flow in Ontario, Canada (Treiman, 1987; Friedman Lentz et al, 1999), in an attempt to elucidate their unusual formation. Terrestrial comparisons were also prominent in the discussions of ALH84001. Several of the observations purportedly serving as evidence of life in the rock were compared with terrestrial counterparts: organically and inorganically grown magnetites (e.g. Chang and Kirshvink, 1989), carbonate rosettes (?), nanofossils (?), etc. While the jury is still out on the life in ALH84001 issue, these sorts of comparisons are critical for learning as much as possible from these small samples from Mars.
5.d. Microscopic observations of petrologic textures
Hawaiian basaltic lava flows are being frozen and sectioned at different points in their cooling history, providing information on the formation and modification of primary petrologic textures within real lava flows (Sharma et al., 2000).
5.e. Mass movement modeling
Evidence exists for various types of landsliding, including debris flows on Mars, however little is known about the slope-forming materials on Mars and the processes that govern their failure. By focusing on a terrestrial debris flow, Bulmer et al. (2001) tested the application of Chezy-type modeling to provide more direct estimates of the dynamics of proposed debris flows on Mars. The model was applied to the General’s slide debris flow in Madison County, Virginia. Topography along and across the debris flow was obtained using GPS equipment. Determinations of velocity were made from observations of superelevation, mudlines, clasts imbedded in trees, vegetation stripping, and H/L. Such an approach is also being used to study the Chaos Jumbles Rock Avalanche, California.
KEY SCIENCE QUESTIONS
The key questions can be divided into 1) Science and 2) Technology/operations areas.
1. The overarching scientific goals of the Mars program have been documented by the Mars Exploration Payload Assessment Group (MEPAG; Greeley, 2001). They include: 1) Detect life or its remnants, 2) Determine present and past climate and its drivers, 3) Determine the geologic processes that have created and modified Mars, and 4) Prepare for human exploration. Underlying this is the fact that we are entering a new phase of Mars study: The era of global mapping is ending and studies will begin to focus on detailed analysis at the regional to outcrop level. This makes it all the more important for Mars geologists of the future to be fully cognizant of geologic processes that operate on Earth which may be analogous to those on Mars.
2. Prediction of capabilities and interpretation of data from future instruments is a critical need. By optimizing the choice of instruments and their operating parameters through tests in analog terrains, we maximize our chances for success. Possible instruments in need of such study include low-frequency airborne or orbital radar sounders; low-frequency airborne or orbital imaging radar; surface ground penetrating radar and EM sounders; seismic instruments; drills; rovers; high resolution stereo imagers from orbit, lander, or microscope; high resolution topography; hyperspectral visible, near-IR, thermal IR; Raman; Mossbauer; SEM/EDAX, Laser-Induced Breakdown Spectroscopy. Accurate georeferencing or geocoding of these data needs to be developed operationally. These instruments should be tested in a variety of sites, listed below. In addition, Mars surface operations, both robotic and human, need to be developed and tested in analog terrains.
RECOMMENDATIONS
As the section on Current State of Knowledge indicates, much Mars analog research has been accomplished and a great deal of activity is currently ongoing. However, more coordination among the many current individual projects could yield additional benefits. In particular, the collection and wide dissemination of terrestrial analog data could be expanded. This could take the form of enhancing already-approved field projects so that additional remote sensing and field measurements are obtained. In addition, data from these campaigns could be archived and made available via CD or the Web to a wider community, allowing broad use of the data. Both additional data collection and its subsequent reduction, calibration, and archiving will require new funding to make it happen.
Focusing the community on a few field sites could also help build the diverse remote sensing data sets and supporting data needed to interpret their equivalents from Mars. This should particularly benefit the technology and operations development community as new instruments and techniques need testing. Laboratory measurements are another area in which ongoing efforts could be enhanced through appropriate funding. Detailed recommendations are given below.
Support for data collection and dissemination for specific Mars analog field sites, however should not preclude science proposals seeking to investigate other field sites, as it is impossible to capture all features and processes of Mars in a small set of sites.
1. Data collection and field observations at terrestrial analog sites.
Coordinated deployment of airborne, spaceborne, field instrumentation, and personnel to several sites should be undertaken to test instruments and technology intended for Mars and to provide data for ongoing studies of terrestrial geologic processes relevant to Mars. Selected scientist-astronauts could participate in field data acquisition to complement their experience in spaceborne observations; they would contribute to the research and develop capabilities for Mars exploration (Dickerson et al., 2000; http://geoinfo.nmt.edu/penguins/home.html)
This is the most important area for support. The support will be used to leverage current investments by NASA codes S (Space Science), Y (Earth Science), and M (Human Exploration); NSF; US Army CRREL; and international agencies by acquiring additional data sets at important analog sites which are being studied for a variety of reasons. These activities should begin as soon as possible in order to benefit current and upcoming Mars missions. An important aspect of these campaigns is the rapid and wide dissemination of data, which should be an explicit part of any data collection under this program.
Costs for this part of the program will probably range from less than $100K for sites requiring only a few field measurements, up to as much as $1M for a coordinated multi-aircraft, spacecraft, and field campaign to Antarctica. These costs should be shared across agencies and it will require significant cross-agency and international coordination to make the larger projects happen.
Desirable field site characteristics include easy access, good infrastructure (power, communication, roads, accommodations, etc.), easy aircraft overflight access, little to no vegetation, cold and/or dry, dominance of a few processes thought to be important on Mars, good documentation (e.g. surficial maps, geologic maps, drill logs, etc.). This work must be accompanied by field mapping and determination of the types, rates, and magnitudes of processes to couple with the interpretation of the instrumental data. Terrestrial geologists familiar with the area should be included in these studies and deployments.
Field sites proposed for initial activities under the Mars analog program:
1.a. Arid regions
Mojave/ Death Valley:
The Mojave Desert/Death Valley region of southeastern California is currently a dry environment but climatic conditions in the past favored wetter conditions. Many examples of dry lake beds (playas) are found in this region which serve as excellent terrestrial analogs to martian basins proposed to contain paleolake deposits and evaporites (Baldridge et al., 2001). As the evidence continues to mount that Mars has experienced warmer and wetter periods throughout its history, areas such as the Mojave Desert and Death Valley become increasingly important as terrestrial analog sites. In addition to the playa deposits, many other geologic processes have affected this region, including tectonism and volcanism which also have implications for the development of the martian highlands. The importance of the southeastern California desert region to understanding Mars is demonstrated by the early field guide developed for this region (Greeley et al., 1978) and the October 2001 workshop on its applicability as a terrestrial analog to the martian highlands (Howard et al., 2001). The clse proximity of NASA’s base of remote sensing aircraft such as AVIRIS, MASTER, and AIRSAR, make data collection even easier.
Central Australia:
Wasson et al. (1988) estimate that the dune fields in Australia represent more than 38% of the world’s aeolian landscape. Although the dune fields are contained in seven interconnected deserts, most dunes are longitudinal, up to 300 km long, 10-35 m high, and spaced 160-200 m apart (Croke, 1997). Twidale (1972) suggests that these dunes originated downwind from debris mounds associated with playas and alluvial plains. Although the dune sand ranges in color from white to dark red, there is an ongoing debate as to whether this is a function of age or sediment source. Understanding sediment sources, transport distances, and ages of dune fields in Australia has implications for understanding similar features on Mars.
A majority of rivers in Australia are ephemeral. Because they are storm-fed, appreciable runoff typically occurs in localized areas within the much larger drainage basin. This results in only portions of the channel or tributaries containing flow at any one time. Streamflow is infrequent, has a rapid onset, and is short-lived (Mabbutt, 1977; Graf, 1988; Bourke and Pickup, 1999). To date, most terrestrial analog studies have focused on the morphologic characteristics associated with perennial rivers or catastrophic floods. However, if martian fluvial systems had abundant sediment supply, were subjected to oscillating climates or base levels, or were formed in an arid environment with high transmission losses, then ephemeral rivers may make better analogs.
MAINTAINING CAPABILITIES FOR THE FUTURE.
1. Continue expanded field workshops and support of projects such as the Houghton Mars Project. Change sites/processes as Mars results come in. Continue building human observational experience and insight by involving astronaut researchers.
2. Continue coordinated deployments of bread-board instruments or instruments similar to those intended for Mars on aircraft, Earth-orbiting spacecraft, and the ground. Develop relationships between NASA offices and other national and international agencies for support of these multi-disciplinary activities. For example, NASA Earth Science may be interested in ice studies or sounding for deep soil moisture; NSF is interested in polar studies as is CRREL. International interest in martian analogs (e.g. CNES, ESA, ASI, etc.) could help support non-US sites in, for example, Africa, Tibet, Atacama Desert, Australia, Iceland, Russia, Canada.
3. Continue support of data archive of terrestrial analog data. Review periodically based on latest Mars results. As Mars materials become better understood, define new Mars analog materials for laboratory study.
4. Establish a few prime sites for continuing study, human training, Education and Public Outreach, etc. (see below)
OTHER
1. Human exploration.
Mars analog studies and geological field training exercises will be crucial in the manned exploration of Mars because of its rich and complex geological history (perhaps even biological history). This will present new and exciting challenges to the human exploration of both the surface and subsurface of Mars. At analog sites, astronauts will be able to carry out the proper field investigations for correct geological context of the landing site as well as the surrounding environs, conduct precision landings, maximize surface mobility, and also perform intelligent sample acquisition and interpretation (Rice, 2000; Dickerson et al., 2000).
At JSC, Shuttle crews are briefed in observing/documenting/interpreting features on Earth that may be analogues for those on Mars. As a result, a growing collection of astronaut-acquired photography is being built, focused on diagnostic attributes of terrestrial sites (e.g., Channeled Scablands) for rigorous comparison with MOC and future images of Mars. An outgrowth of early efforts was an online education/outreach publication produced in collaboration with planetary scientists at JSC and LPI (Willis et al., 2000).
On Devon Island, a number of studies are currently underway under the auspices of the NASA Haughton-Mars Project to develop some of the technologies, strategies and human factors experience that will help plan the future exploration of Mars and other planets by robots and humans (www.marsonearth.org). The HMP is established as an international interdisciplinary field research project managed for NASA by the private SETI Institute, thus offering an opportunity at the same time to explore government-private partnerships in extracting the scientific and technological potential of Mars analog research.
2. Education and Public Outreach.
For this application, the visual aspect of the analog site and the logistics and accessibility are the most important factors. The more accessible sites especially could become “standard” sets for filmed news stories and documentaries. Demonstrations of rovers are always popular- more so in a Mars-like setting. The public can be encouraged to visit some of the sites, yielding an opportunity to describe how the site is like Mars and how it isn’t. National Monuments and Parks such as Death Valley and Mojave Desert, may be interested in helping to support this. Hawaii Volcanoes National Park already hosts annual workshops for teachers and school students who use the volcanic terrain as a planetary analog. Planning for manned and un-manned surface activities on the planets (including Mars) are included in the program.
References
Abdelsalam, M. G., C.A. Robinson, F. El-Baz and R.J. Stern, 2000, Applications of Orbital Imaging Radar for Geologic Studies in Arid Regions: The Saharan Testimony, PE&RS, v. 66, No. 6, p. 717-726.
Abdelsalam, M.G., R.J. Stern, 1996, Mapping Precambrian structures in the Sahara Desert with SIR-C/X-SAR radar: The Neoproterozoic Keraf Suture, NE Sudan, J. Geophys. Res., v. 101, p. 23063-23076.
Abrams, M.J., E. Abbott, A. Kahle, 1991, Combined use of visible, reflected infrared, and thermal infrared images for mapping Hawaiian lava flows, J. Geophys. Res., v. 96, p. 475-484.
Agrinier et al., 2001, Fast back-reactions of shock-released CO2 from carbonates: An experimental approach, Geochim. Cosmochim. Acta, v. 65, p.2615-2632.
Allen, C. C., Westall, F., Schelble, R. 2001. Importance of a Martian hematite site for Astrobiology, Astrobiology, v. 1, p. 111-123.
Anderson, F.S., M.H. Hecht, F.D. Carsey, P.G. Conrad, W.F. Zimmerman, L.C. French, H. Engelhardt, 2001, In-Situ ice core analysis of Longyearbreen Glacier using a cryobot: Preparation for the northern polar cap of Mars, abs. Eos Trans. AGU, v. 82.
Arvidson, R.E., M.K. Shepard, E.A. Guinness, S.B. Petroy, J.J. Plaut, D.L. Evans, T.G. Farr, R. Greeley, N. Lancaster, L.R. Gaddis, 1993, Characterization of lava-flow degradation in the Pisgah and Cima volcanic fields, California, using Landsat Thematic Mapper and AIRSAR data, Geol. Soc. Amer. Bull., v. 105, p. 175-188.
Baker, V. R., 1981, Australian analogs to geomorphic features on Mars: Reports of the
Planetary Geology Program – 1981, NASA Tech. Memo. 84211, p. 329-330.
Baker, V. R. and D. Nummedal, 1978, The Channeled Scabland, NASA Planetary Geology Program, Washington, D.C.
Baldridge, A.M., J.D. Farmer, J.E. Moersch, 2001, Remote sensing of terrestrial analogs for evaporite basins on Mars: Analysis of ground truth, abs. Eos Trans. AGU, v. 82.
Behrendt, J.C., D. D. Blankenship, D. L. Morse, and R. E. Bell, 2001, Removal of subglacially erupted volcanic edifices beneath the divide of the West Antarctic Ice Sheet interpreted from aeromagnetic and radar ice sounding surveys, in J. A. Gamble, D. N. B. Skinner, S. Henrys, and R. Lynch, eds., Proceedings Volume 8th International Symposium on Antarctic Earth Sciences, Wellington, p. (in press).
Behrendt, J.C., D. D. Blankenship, C. A.Finn, R. E. Bell, R.E. Sweeney, S. M. Hodge, and J. M. Brozena, 1994, CASERTZ aeromagnetic data reveal late Cenozoic flood basalts in the West Antarctic rift system, Geology, v. 22, p. 527-530.
Behrendt, J.C., Finn, C.A., Blankenship, D.D., and Bell, R.E., 1998, Aeromagnetic evidence for a volcanic caldera complex beneath the divide of the West Antarctic Ice Sheet, Geophysical Research Letters, v. 25, p. 4385-4388.
Berthelier J.-J, R. Ney, F. Costard, M. Hamelin, A. Meyer, B. Martinat, A. Reineix, Th. Hansen, M. Bano, W. Kofman, F. Lefeuvre, Ph. Paillou, 2000, The GPR Experiment on Netlander, Planetary and Space Science, v. 48, p. 1161-1180.
Blankenship, D.D., R.E. Bell, S.M. Hodge, J.M. Brozena, J.C. Behrendt, and C.A. Finn, 1993, Active volcanism beneath the West Antarctic ice sheet and implications for ice-sheet stability, Nature, 361, p. 526-529.
Blankenship, D.D., D.L. Morse, C.A.Finn, R.E. Bell, , M.E. Peters, S. E. Kempf, S. M. Hodge, M. Studinger, J. C. Behrendt, and J. M. Brozena, 2001, Geologic controls on the initiation of rapid basal motion for West Antarctic ice streams: A geophysical perspective including new airborne radar sounding and laser altimetry results, in R. B. Alley, and R. A. Bindschadler, eds., The West Antarctic Ice Sheet: Behavior and Environment, v. 77, Antarctic Research Series, Washington, D.C., American Geophysical Union, p. 105-121.
Bourke and Zimbelman, 2001, (Craddock) ***
Chang S.B.R. and J. L. Kirshvink, 1989, Magnetofossils, the magnetization of sediments, and the evolution of magnetite biomineralization, Annual Rev. Earth Planet. Sci. v. 117, p. 169-195
Chapman, M. G., 2001, Layered, massive, and thin sediments on Mars: Possible Late Noachian to Late Amazonian tephra?, in Chapman, M. G. and Smellie, J. L., editors, Volcano/Ice Interactions on Earth and Mars: London, The Geological Society, special volume, in press.
Chapman, M., and others, 2000, Volcano-ice interactions on Earth and Mars, Reykjavik, Iceland, http://wwwflag.wr.usgs.gov/USGSFlag/Land/IcelandMeeting.
Clifford, S., 2000, The Second International Conference on Mars Polar Science and Exploration, Iceland, http://www.lpi.usra.edu/meetings/polar2000
Cockell, C. S., and P. Lee 2001. The biology of terrestrial impact craters: A review. Biological Reviews. In press.
Cockell, C. S., P. Lee, A. C. Schuerger, L. Hidalgo, J. A. Jones, and M. D. Stokes 2001. Microbiology and vegetation of micro-oases and polar desert, Haughton impact crater, Devon Island, Canada. Arctic Antarct. Alpine Res. 22, 306-318.
Craddock, R.A., T. A. Maxwell, and A. D. Howard, 1997, Crater morphometry and modification in the Sinus Sabaeus and margaritifer Sinus regions of Mars, J. Geophys. Res., v. 102, p. 13,321-13,340.
Dann J.C., A. H. Holzheid, T. L. Grove, and H. Y. McSween, Jr., 2001, Phase equilibrium of the Shergotty meteorite, Constraints on pre-eruptive H2O contents of martian magmas and fractional crystallization under hydrous conditions. Meteorit. Planet. Sci. v. 36, p.793-806.
Dickerson, P. W., Muehlberger, W.R., and Bauer P.W., 2000, Astronaut training in field geophysical methods, Albuquerque, AIAA Space 2000 conference proceedings.
Durham W. B. et al.,1999a, Abstracts, Intl. Workshop on the Martian Ice Caps, Copenhagen.
Durham W. B. et al. 1999b, Geophys. Res. Lett. v. 26, p. 3493-3496.
Durham, W.B., S. H. Kirby, and L. A. Stern, 2000, Comparative Rheologies of Solid H2O, CO2, and CO2 Clathrate Hydrate. Second International Conference on Mars Polar Science and Exploration, Reykjavik, Iceland.
Edgett, K.E., J.W. Rice, Jr., and V.R. Baker (ed. by): Field trips accompanying the Mars Pathfinder Landing Site II workshop, Channeled Scabland and Lake Missoula break-out areas in Washington and Idaho, Lunar and Planetary Institute Tech. Rept., 95-01, p. 63.
El-Baz, F. and T. A. Maxwell, Desert Landforms of Southwest Egypt: A Basis for Comparison with Mars, NASA CR-3611, Washington, D.C., 1982.
El-Baz, F. C.A. Robinson, M.M. Mainguet, M. Said, M. Nabih, I.H. Himida, H.A. El-El-Etr, 2001, Distribution and morphology of palaeo-channels in southeastern Egypt and northwestern Sudan, Palaeoecology of Africa (Klaus Heine, editor), v 27, p. 239-258.
Evans, D.E., C. Weitz, 1999, Workshop on the weathering of basalts on Earth and Mars, ***
Evans, C.E., 2001, Astronauts photograph Mt. Pinatubo: NASA, Earth Observatory, June 15, 2001, http://earthobservatory.nasa.gov/Study/AstronautPinatubo/
Farr, T.G., 1992, Microtopographic evolution of lava flows at Cima volcanic field, Mojave Desert, California, J. Geophys. Res., v. 97, p. 15171-15179.
Farr, T.G. and J.B. Adams, 1984, Rock coatings in Hawaii, Geological Society of America Bulletin, v. 95, p. 1077-1083.
Foessel, A., and P. Lee 2001. Ground Penetrating Radar deployment in Mars-like environment. ISAIRAS Conf., 2001.
Fredriksson, K., A. Dube, D. J. Milton, and M. S. Balasundaram, 1973, Lonar Lake, India: An impact crater in basalt, Sci. v. 180, p. 862-864.
Friedman Lentz R.C., G. J. Taylor, and A. H. Treiman , 1999, Formation of a martian pyroxenite: A comparative study of the nakhlite meteorites and Theo’s Flow, Meteorit. Planet. Sci. v. 34, p. 919-932.
Fudali, R.F., 1973, Roter Kamm, Evidence for an impact origin, Meteoritics, v. 8, p. 245-
257.
Glass, B. J. and P. Lee, 2001, Airborne geomagnetic investigations at the Haughton Impact Structure, Devon Island, Nunavut, Canada, Lunar Planet. Sci., XXXII, Abstract #2155.
Golombek, M. P., K. S. Edgett, and J. W. Rice, 1995, Mars Pathfinder Landing Site Workshop II: Characteristics of the Ares Valles Region and Field Trips in the Channeled Scabland, Washington, LPI Tech. Rpt. No. 95-01, Lunar and Planetary Institute, Houston, TX.
Gooding J.L., S. J. Wentworth and M. E. Zolensky, 1991, Aqueous alteration of the Nakhla meteorite. Meteoritics v. 26, p. 135-143.
Grant, J.A., Koeberl, C., Reimold, W.U., Schultz, P.H., Brandt, D., 1997, Degradation history of the Roter Kamm impact crater, Namibia: J. Geophys. Res. v. 102, p. 16,327-16,388.
Grant, J.A., and Schultz, P.H., 1994, Erosion of ejecta at Meteor Crater: Constraints from ground penetrating radar, in GPR ’94: Proceedings of the Fifth International Conference on Ground Penetrating Radar, June 12-16, 1994, University of Waterloo, Kitchener, Ontario, Canada, p. 789-803.
Grant, J.A. 1990, Evaluating the Evolution of Process Specific Degradation Signatures Around Impact Craters: Int. J. Impact Engineering, v. 23, p. 331-340.
Grasby, S. E., Allen, C. C., Longazo, T., Lisle, J. T. 2001, Abstract, Geological Society America, Boston, November 1-10, 2001, p. A451
Greeley, R., 2001, Science Planning for Exploring Mars: part 2, Scientific goals, objectives, investigations, and Priorities, JPL Pub. 01-7.
Greeley, R., 1974, Hawaiian Planetology Conference, NASA TMX 62362, Washington, D.C.
Greeley, R. and J. S. King, 1977, Volcanism of the Eastern Snake River Plain, Idaho, A Comparative Planetary Geology Guidebook, NASA Office of Planetary Geology, Washington, DC.
Greeley, R., M. B. Womer, R. P. Papson, and P. D. Spudis, 1978, Aeolian Features of Southern California, A Comparative Planetary Geology Field Guide, NASA Office of Planetary Geology, Washington, D.C.
Grieve, R.A.F., C. A. Wood, J. B. Garvin, G. McLaughlin, J. F McHone, Jr., 1988, Astronaut’s guide to terrestrial impact craters. LPI Technical Report Number 88-03, Houston, Texas, p. 89.
Hamilton V.E., M. B. Wyatt, H. Y. McSween, Jr., P. R. Christensen, 2001, Analysis of terrestrial and martian volcanic compositions using thermal emission spectroscopy: Application to martian surface spectra from the Mars Global Surveyor Thermal Emission Spectrometer. JGR, v. 106, p. 14733-14746.
Heggy, E.., Ph. Paillou, G. Ruffié, J.-M. Malézieux, F. Costard, G. Grandjean, 2001, On water detection in the Martian subsurface using sounding Radar, Icarus, (accepted).
Holt, J.W., 2001, Airborne Surveys Conducted by SOAR for Geologic Studies in Antarctica, 1998-2001, Eos Trans. AGU, v. 82, p. 20.
Hon, K., J. Kauahikaua, R, Denlinger, and K. Mackay, 1994, Emplacement and inflation of pahoehoe sheet flows: Observations and measurements of active alva flows on Kialuea Volcano, Hawaii, Geol. Soc. Am. Bul., v. 106, p. 351-370.
Horz, F., 1982, Ejecta of the Ries Crater, Germany, GSA Special Paper 190.
Howard, A.D., J. Moore, J. Rice, 2001, Field trip and workshop on the Martian Highlands and Mojave Desert analogs, http://www.lpi.usra.edu/meetings/martianhighlands2001.
Howard, A. D., R. C. Kochel, and H. E. Holt, 1988, Sapping Features of the Colorado Plateau, A Comparative Planetary Geology Field Guide, NASA SP-491, Washington, D.C.
Kapitsa, A.P., J. K. Ridley, G. de Q. Robin, M. J. Siegert, and I. A. Zotikov, 1996, A Large Deep Freshwater Lake Beneath the Ice of Central East Antarctica, Nature, v. 381, p. 684-686.
Kargel, J. S., J. Moore, and T. Parker, 1993, Workshop on the Martian Northern Plains, Sedimentological, Periglacial, and Paleoclimatic Evolution, LPI Tech. Rpt. No. 93-04, Lunar and Planetary Institute, Houston, TX.
Keszthelyi, L, A. S. McEwen, Th. Thordarson, 2000, Terrestrial analogs and thermal models for Martian flood lavas, J. Geophys. Res., v. 105, p. 15027-15049.
Laity, J. E. and D. C. Pieri, 1980, Sapping processes in tributary valley systems, NASA Tech. Memo 81776, p. 271-273,
Lanagan, P.D., A. S. McEwen, L. P. Keszthelyi, Th. Thordarson, 2001, Rootless cones on Mars indicating the presence of shallow equatorial ground ice in recent times, Geophys. Res. Lett., v.28, p. 2365-2367.
Larsen, J. and D. Dahl-Jensen, 2000, Interior Temperatures of the Northern Polar Cap on Mars, Icarus, v. 144, p. 256-462.
Lee, P., C. S. Cockell, M. M. Marinova, C. P. McKay, and J. W. Rice, Jr. 2001. Snow and Ice melt slope flow features on Devon Island, Nunavut, Arctic Canada, as possible analogs for recent slope flow features on Mars. Lunar Planet. Sci. Conf. XXXII, Mar, 2001.
Lee, P. 2000. Selective fluvial erosion on Mars: Glacial selective linear erosion on Devon Island, Nunavut, Arctic Canada, as a possible analog. Lunar & Planet. Sci. Conf. XXXI, Mar, 2000.
Lee, P., and J. W. Rice, Jr. 1999. Small valley networks on Mars: The glacial meltwater channel networks of Devon Island, Nunavut Territory, Arctic Canada, as possible analogs. 5th Mars Conference, Jul 1999.
Lee, P., J. W. Rice, Jr., T. E. Bunch, R. A. F. Grieve, C. P. McKay, J. W. Schutt, and A. P. Zent 1999. Possible analogs for small valleys on Mars at the Haughton impact crater site, Devon Island, Canadian High Arctic. Lunar Planet. Sci. Conf. XXX., Mar 99.
Lee, P., T. E. Bunch, N. Cabrol, C. S. Cockell, R. A. F. Grieve, C. P. McKay, J. W. Rice, Jr., J. W. Schutt, and A. P. Zent 1998. Haughton-Mars 97 – I: Overview of observations at the Haughton impact crater, a unique Mars analog site in the Canadian High Arctic. Lunar Planet. Sci. Conf. XXIX, Mar 98, 1973-1974.
Lee, P. 1997. A unique Mars/Early Mars analog on Earth: The Haughton impact structure, Devon Island, Canadian Arctic. In Conf. on Early Mars: Geologic and hydrologic evolution, physical and chemical environments, and the implications for life. LPI Contrib. No. 916, 50.
Lee, P. 1993. Briny lakes on early Mars? Terrestrial intracrater playas and martian candidates. LPI Tech. Rep. 93-03, Part 1, 17.
Lentz R.C.F., H. Y. McSween H.Y. Jr., J. G. Ryan and L. R. Riciputi, 2001, Water in martian magmas, Clues from light lithophile elements in shergottite and nakhlite pyroxenes, Geochim. Cosmochim. Acta 65, (in press).
Leshin L. A., 2000, Insights into martian water reservoirs from analysis of martian meteorite QUE94201. GRL v. 27 (14), p. 2017-2020.
McCauley, J.F., Breed, C.S., Schaber, G.G., McHugh, W.P., Issawi, B., Haynes, C.V., Grolier, M.J. and Kilani, A.E., 1986, Paleodrainage of the Eastern Sahara – the radar rivers revisited, IEEE Trans. Geosci. Remote Sens., v. 24, p. 624-648.
Macdonald, G. A, 1953, Pahoehoe, aa, and block lava. Am. J. Sci., v. 251, p. 169-191.
McEwen, A., D. Burr, J. Hardardottir, A. Hoskuldsson, L. Keszthelyi, P. Lanagan, A. Snorrason, T. Thordarson, 2001, Icelandic analogs for volcanic and fluvial processes on Mars, abs. Eos Trans. AGU, v. 82.
McHone, J.F, R. Greeley, K.K. Williams, D.G. Blumberg, and R.O. Kuzmin 2002, Space Shuttle observations of terrestrial impact structures using SIR-C and X-SAR radars, accepted by Meteoritics and Planetary Sciences.
McSween , H.Y., Jr., T. L. Grove, R.C.F. Lentz, J. C. Dann, A. H. Holzheid, L. R. Riciputi, J. G. Ryan, 2001, Geochemical evidence for magmatic water within Mars from pyroxenes in the Shergotty meteorite. Nature v. 204, p. 487-490.
Martinez et al., 1994, A SEM-TEM and stable isotope study of carbonates from the Haughton impact structure, Canada, EPSL v. 121, p. 559-574.
Masaitis, V. L. ,1976, Astroblemes in the USSR. Intern. Geol. Rev.,v. 18, p. 1249-1258.
Mege, D. and S. P. Reidel, 2001, A method for estimating 2D wrinkle ridge strain from application of fault displacements scaling to the Yakima folds, Washington, Geophysical Research Letters, v. 28, p. 3545-3548.
Mege, D. and R. E. Ernst, 2001, Contractional effects of mantle plumes on Earth, Mars, and Venus: in R. E. Ernst, and K. L. Buchan, eds., Mantle Plumes: Their Identification Through Time, Boulder, Colorado, Geological Society of America Special Paper, v. 352, p. 103-140.
Milton, D. J., 1968, Structural Geology of the Henbury Meteorite Craters Northern Territory, Australia, U.S. Geological Survey Professional Paper 599-c, p. 17.
Moersch, J. E, J. Farmer, and A. Baldridge, 2001, Remote Sensing of Evaporite Minerals in Badwater Basin, Death Valley, at Varying Spatial Scales and in Different Spectral Regions, in Howard et al., 2001.
Morris, P.A., S.J. Wentworth, K. Thomas-Keprta, J.L. Lisle, C.C. Allen, D.S. McKay, 2000, Dead Sea microbes, Fossilization and potential biomarkers, abs. Geol. Soc. Amer., Reno, NV.
Morse, D.L., E. D. Waddington, and E. J. Steig, E.J., 1998, Ice Age Storm Trajectories Inferred from Radar Stratigraphy at Taylor Dome, Antarctica, Geophysical Research Letters, v. 25 (17), p. 3383-3386.
Morse, D.L., D.D. Blankenship, E D. Waddington, and T.A. Neumann, 2001, Candidate Sites for Deep Ice Coring in West Antarctica: Results from Aerogeophysical Surveys and Thermo-kinematic Modeling, Annals of Glaciology, v. 35, (in press).
Ori, G.G., G. Komatsu, L. Marinangeli, ed., 2001, Field trip guidebook for Workshop on Exploring Mars Surface and its terrestrial analogue, p. 95, http://irsps.sci.unich.it/education/tunisia
Osinski, G.R., J.G. Spray, P. Lee, 2001, Impact-induced hydrothermal activity within the Haughton impact structure, Arctic Canada: Generation of a transient, warm, wet oasis, Meteoritics & Planetary Science, v. 36, p. 731-745.
Oswald, G.K.A., and Robin, G. de Q., 1973, Lakes Beneath the Antarctic Ice Sheet, Nature, v. 245 (5423), p. 251-254.
Paillou, Ph., G. Grandjean, J.-M. Malézieux, G. Ruffié, E. Heggy, D. Piponnier, P. Dubois, J. Achache, 2001, Performances of Ground Penetrating Radars in Arid Volcanic Regions: Consequences for Mars Subsurface Exploration, Geophysical Research Letters, v. 28, p. 911-914.
Peters, M.E., D. D. Blankenship, D. L. Morse, 2001, A New Radar Sounding Method for Improving the Characterization of Subglacial Interfaces Applied to Ice Streams B and C, West Antarctica, Journal of Glaciology, (in press).
Peterson, D. W. and R. I. Tilling, 1980, Transition of basaltic lava from pahoehoe to aa, Kilauea Volcano, Hawaii, Field observations and key factors, J. Volcanol. Geotherm. Res., v. 7, p. 271-293.
Rhodes, J.M, and J. P. Lockwood (editors) Mauna Loa Revealed: Structure, Composition, History, and Hazards, AGU Geophysical Monograph, v. 92, p. 348.
Rice, J R. Jr., 2000, J. E. Concepts and Approaches for Mars Exploration, 2000, Houston, Texas
Rice, J.W. Jr, 2001, High Latitude Terrestrial Lacustrine and Fluvial Field Analogs for the Martian Highlands in Howard et al.,2001.
Rice, J.W. Jr., A. J. Russell, F. S. Tweed, Ó. Knudsen, M. J. Roberts, P. M. Marren R. I. Waller, E. L. Rushmer, H. Fay, and T. D. Harris, 2000, Field Investigations of Icelandic Jökulhlaups as an Analog to Floods on Mars, Second International Conference on Mars Polar Science and Exploration, Reykjavik, Iceland.
Robertson, P.B., 1988, The Haughton impact structure, Devon Island, Canada, Setting and history of investigations, Meteoritics, v. 23, p. 181-184.
Robinson, C.A., F. El-Baz and V. Singhroy, 1999, Subsurface Imaging By Radarsat, Comparison with Landsat TM Data and Implications to Ground Water in the Selima Area, Northwestern Sudan, Canadian Journal of Remote Sensing, v. 25, (3), p. 268-277.
Robinson, C.A., F. El-Baz, M. Ozdogan, M. Ledwith, D. Blanco, S. Oakley, and J. Inzana, 2000, Use of Radar Data to Delineate Palaeodrainage Flow Directions in the Selima Sand Sheet, Eastern Sahara, PE&RS, v. 66, (6) p. 745-753
Roddy, D J., 1978, Pre-impact geologic conditions, physical properties, energy calculations, meteorite and initial crater dimensions and orientations of joints, faults, and walls at Meteor Crater, Arizona: Proc. Lunar and Planet. Sci. Conf., v. 9, p. 3891-3930.
Schaber, G., J.F. McCauley, C. S. Breed, G. R. Olhoeft, 1986, Shuttle Imaging Radar, Physical controls on signal penetration and subsurface scattering in the Eastern Sahara, IEEE Transactions on Geoscience and Remote Sensing, v. GE-24, (4) p. 603-623.
Schaber, G.G., McCauley, J.F. and Breed, C.S., 1997. The use of multifrequency and polarimetric SIR-C/X-SAR data in geologic studies of Bir Safsaf, Egypt, Remote Sensing and Environment, v, 59, p. 337-363.
Self, S., L. Keszthelyi, and Th. Thordarson, 1998, The importance of pahoehoe. Annu. Rev. Earth. Planet. Sci., v. 26, p. 81-110.
Sharma K, L. Keszthelyi, C. Thornber, S. Self , 2000, Variations in the cooling, crystallization textures, and glass chemistry during emplacement of Kilauea pahoehoe lobes, as constrained by in situ field experiments. GSA Annu. Meeting, Online Abstracts.
Siegert, M J., J.A. Dowdeswell, M. R. Gorman, and N. F. McIntyre, 1996, An Inventory of Antarctic Sub-glacial Lakes, Antarctic Science, v. 8 (31), p. 281-286.
Stern, R.J. and Abdelsalam, M.G., 1996. The origin of the Great Bend of the Nile from SIR-C/X-SAR Imagery, Science, v. 274, p. 1696-1698.
Stoker, C.R., N. Cabrol, T. Roush, J. Moersch, et al., 2001, The 1999 Marsokhod Rover Mission Simulation at Silver Lake California: Mission Overview, Data Sets, and Summary of Results J. Geophys. Res., v. 106, p. 7639-7663.
Swindle T.D., J.A. Grier, B. Li, E.D. Olsen, D.J. Lindstrom and A.H. Treiman, 1997, K-Ar ages for the near-surface liquid water on Mars in the last few hundred million years. (abs) Lunar and Planet. Sci. XXVIII, p. 1403-1404.
Thordarson, Th., and Self, S., 1993, The Laki (Skaftár Fires) and Grímsvötn eruptions in 1783-1785, Bull. Volcanol., v. 55, p. 233-263.
Thorarinsson, S., 1953, The Crater Groups in Iceland, Bull. Volcanol., v. 14, p. 3-44.
Treiman A.H., 1987, Geology of the nakhlite meteorites, Cumulate rocks from flows and shallow intrusions (abs.) Lunar Planet. Sci. v. 18, p. 1022-1023.
Wadhwa, M., 2001, Redox state of Mars’ upper mantle and crust from Eu anomalies in Shergottite pyroxenes. Sci. v. 291, p. 1527-1530.
Wall, S.D., T. G. Farr, J.-P. Muller, P. Lewis and F.W. Leberl, 1991, Measurement of surface microtopography, Photogramm. Eng. Rem. Sens., v. 57, p. 1075-1078.
Watters, T. R. and M. P. Golombek, 1989, MEVTV Workshop on Tectonic Features on Mars, LPI Tech. Rpt. No. 89-06, Lunar and Planetary Institute, Houston, TX.
Wentworth, S.J., P. A. Morris, 2001, The geology, paleontology, and biology of evaporite and near-evaporite system in both terrestrial and extraterrestrial environments, Topical Session 27, Geological Society of America, Boston, November 1-10, 2001.
Westall, 2001 ***
Williams, K.K. and R. Greeley 2001, Radar attenuation by sand: Laboratory measurements of radar transmission, IEEE Trans. Geoscience Remote Sensing, v. 39, p. 2521-2526.
Williams, K.K. and R. Greeley 2001, Laboratory measurements of radar transmission through dust with implications for radar imaging on Mars, In Lunar and Planetary Science XXXII, Abstract #1264, Lunar and Planetary Institute, Houston (CD-ROM).
Willis, K., P. W. Dickerson, B. H. McRay, 2000, Canyons, Craters and Drifting Dunes – Terrestrial Analogues on Earth’s Moon and Mars, JSC, http://eol.jsc.nasa.gov/newsletter/planetary/sld001.htm.
World Crater Inventory, http://www.unb.ca/passc/inventory/
Wyatt M. B., V. E. Hamilton, H. Y. McSween Jr., P. R. Christensen, and L. A. Taylor, 2001, Analysis of terrestrial and martian volcanic compositions using thermal emission spectroscopy: 1. Determination of mineralogy, chemistry, and classification strategies. JGR, v. 10, p. 14711-14732.
Zuber, M.T., and 20 colleagues ,1998, Observations of the north polar region of Mars from the Mars Orbiter Laser Altimeter, Science, v. 282, p. 2053-2060.
Contributors:
Tom G. Farr (Jet Propulsion Laboratory)
Steve Arcone (Cold Regions Research and Engineering Laboratory)
Ray Arvidson (Washington University)
Vic Baker (University of Arizona)
Nadine Barlow (University of Central Florida)
David Beaty (Jet Propulsion Laboratory)
Mary Sue Bell (Johnson Space Center)
Donald Blankenship (University of Texas Institute for Geophysics)
Nathan Bridges (Jet Propulsion Laboratory)
Geoff Briggs (Ames Research Center)
Mark Bulmer (JCET University of Maryland Baltimore County)
Frank Carsey (Jet Propulsion Laboratory)
Steve Clifford (Lunar and Planetary Institute)
Bob Craddock (National Air and Space Museum)
Patricia W. Dickerson (Johnson Space Center)
Natalia Duxbury (Jet Propulsion Laboratory)
Gillian Galford (Washington University)
Jim Garvin (NASA Headquarters)
John Grant (National Air and Space Museum)
Jacklyn R Green (Jet Propulsion Laboratory)
Tracy K.P. Gregg (University at Buffalo)
Ed Guinness (Washington University)
Vicki Hansen (Southern Methodist University)
Michael H Hecht (Jet Propulsion Laboratory)
John Holt (University of Texas Institute for Geophysics)
Alan Howard (University of Virginia)
Laszlo Keszthelyi (University of Arizona)
Pascal Lee (SETI Institute)
Peter Lanagan (University of Arizona)
Rachel Lentz (University of Tennessee)
David Leverington (National Air and Space Museum)
Lucia Marinangeli (International Research School of Planetary Sciences)
Jeffrey E. Moersch (University of Tennessee)
Penny Morris-Smith (Johnson Space Center)
Peter Mouginis-Mark (University of Hawaii)
Gary Olhoeft (Colorado School Mines)
Gian G. Ori (International Research School of Planetary Sciences)
Philippe Paillou (University of Bordeaux)
James Reilly (Johnson Space Center)
Jim Rice (Arizona State University)
Cordula Robinson (Boston University)
Mike Sheridan (University at Buffalo)
Kelly Snook (Ames Research Center)
Brad Thomson (Brown University)
Kevin Watson (Johnson Space Center)
Kevin Williams (Arizona State University)
Kenji Yoshikawa (University of Alaska Fairbanks)